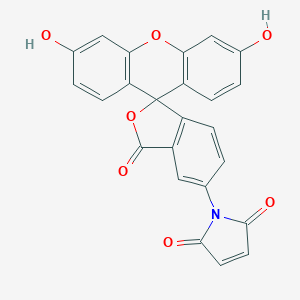
Fluorescein-5-maleimide
Vue d'ensemble
Description
Fluorescéine-5-maléimide: est un colorant fluorescent largement utilisé en recherche biochimique. Il est connu pour sa capacité à réagir avec les groupes thiol, ce qui en fait un outil précieux pour le marquage des protéines et d'autres molécules contenant des groupes sulfhydryles libres. Le composé a une longueur d'onde d'excitation de 494 nm et une longueur d'onde d'émission de 518 nm, ce qui le rend adapté à diverses applications basées sur la fluorescence .
Méthodes De Préparation
Voies de synthèse et conditions de réaction: La fluorescéine-5-maléimide est synthétisée en faisant réagir la fluorescéine avec l'anhydride maléique. La réaction se produit généralement dans un solvant organique tel que le diméthylformamide (DMF) ou le diméthylsulfoxyde (DMSO) dans des conditions douces. Le groupe maléimide du composé réagit de manière optimale avec les groupes sulfhydryles à un pH de 6,5 à 7,5, formant une liaison thioéther stable .
Méthodes de production industrielle: En milieu industriel, la production de fluorescéine-5-maléimide implique une synthèse à grande échelle utilisant des conditions de réaction similaires à celles des milieux de laboratoire. Le processus comprend des étapes de purification telles que la recristallisation et la chromatographie pour garantir une pureté et un rendement élevés du produit final .
Analyse Des Réactions Chimiques
Types de réactions: La fluorescéine-5-maléimide subit principalement des réactions de substitution avec les groupes thiol. Le groupe maléimide est très réactif envers les groupes sulfhydryles libres, formant des liaisons thioéther stables. Cette réaction est très spécifique et se produit dans des conditions douces, ce qui la rend adaptée au marquage de molécules biologiques sensibles .
Réactifs et conditions courants:
Réactifs: Fluorescéine-5-maléimide, molécules contenant des thiols (par exemple, résidus cystéine dans les protéines)
Conditions: pH 6,5 à 7,5, température ambiante, solvants organiques tels que le DMF ou le DMSO
Principaux produits: Le principal produit de la réaction entre la fluorescéine-5-maléimide et les molécules contenant des thiols est un conjugué où le colorant fluorescéine est lié de manière covalente à la molécule cible via une liaison thioéther .
Applications De Recherche Scientifique
Protein Labeling and Tracking
F5M is primarily used for labeling proteins to track their interactions and dynamics in biological systems. It reacts specifically with cysteine residues, which can be either naturally occurring or introduced through site-directed mutagenesis. This specificity allows researchers to study protein conformations and interactions without interfering with other amino acids like histidine or methionine .
Case Study: Cysteine Residue Accessibility
A study demonstrated the use of F5M to analyze the solvent accessibility of cysteine residues in viral-like particles (VLPs). The labeling reaction allowed for the identification of reactive cysteines on the surface of VLPs, providing insights into their structural properties under native conditions .
Biosensors Development
F5M has been employed in the development of peptide-based fluorescence biosensors for detecting engineered nanoparticles (ENPs). In one study, a peptide biosensor was constructed using F5M to monitor interactions between peptides and negatively charged ENPs. The changes in fluorescence intensity were correlated with nanoparticle concentration, offering a rapid detection method .
Table 1: Summary of Biosensor Applications Using F5M
Quality Control in Bioprocessing
F5M is utilized in quality control assays for monoclonal antibody production. A surface plasmon resonance (SPR)-based assay incorporated F5M to quantify antibodies produced in mammalian cell cultures. This method allowed for real-time monitoring of antibody bioactivity and quality throughout the production process .
Mécanisme D'action
Fluorescein-5-maleimide exerts its effects by reacting with free sulfhydryl groups on target molecules. The maleimide group in the compound forms a stable thioether bond with the thiol group, resulting in the covalent attachment of the fluorescein dye to the target molecule. This labeling allows for the visualization and tracking of the target molecule using fluorescence-based techniques .
Comparaison Avec Des Composés Similaires
Composés similaires:
5-Iodoacétamido-fluorescéine: Un autre colorant fluorescent réactif aux thiols qui réagit avec les groupes sulfhydryles pour former des liaisons thioéther stables.
N-(2-Aminoéthyl)maléimide: Un dérivé de maléimide qui réagit avec les groupes thiol mais qui ne possède pas les propriétés fluorescentes de la fluorescéine-5-maléimide.
Unicité: La fluorescéine-5-maléimide est unique en raison de sa combinaison de forte réactivité envers les groupes thiol et de ses propriétés fluorescentes. Cela en fait un outil idéal pour le marquage et l'étude des molécules biologiques dans diverses applications de recherche .
Activité Biologique
Fluorescein-5-maleimide (F5M) is a fluorescent dye extensively utilized in bioconjugation and bioimaging applications due to its ability to react specifically with thiol groups in proteins. This article explores the biological activity of F5M, focusing on its mechanisms of action, applications in biosensing, and its role in various research studies.
This compound is characterized by its maleimide group, which selectively reacts with free thiol groups (-SH) present in cysteine residues of proteins. This reaction forms stable thioether bonds, making F5M a powerful tool for labeling proteins without altering their functional properties. The chemical structure can be represented as follows:
The reaction mechanism involves the nucleophilic attack of the thiol group on the maleimide carbon, leading to the formation of a stable conjugate. This specificity allows for targeted labeling of proteins, facilitating studies on protein interactions and dynamics.
Applications in Biosensing
F5M has been effectively employed in biosensing applications, particularly for detecting biomolecules and monitoring enzymatic activity. One notable study demonstrated the use of fluorescein-labeled β-lactamase mutants for antibiotic detection. The fluorescence intensity varied significantly upon substrate binding, indicating successful biosensing capabilities:
- Fluorescence Enhancement : The binding of β-lactam antibiotics to the fluorescein-labeled enzyme resulted in an increase in fluorescence, which was quantitatively measured and correlated with antibiotic concentration .
- Mechanism : Upon binding, fluorescein moved from a buried hydrophobic region to a more solvent-exposed area, leading to enhanced fluorescence signal .
1. Detection of Antibiotics
A study involving the PenP β-lactamase mutant E166Cf highlighted the effectiveness of F5M in biosensing applications. The modified enzyme displayed a significant increase in fluorescence when interacting with penicillin G, showcasing its potential as a biosensor for antibiotic detection .
Parameter | Value |
---|---|
Enzyme | PenP E166Cf |
Substrate | Penicillin G |
Fluorescence Increase | Rapid enhancement within 40 seconds |
Detection Method | Mass spectrometry |
2. Protein Interaction Studies
This compound has also been utilized to study protein interactions through fluorescence anisotropy. In one experiment, glutathione S-transferase (GST) was conjugated with F5M, allowing researchers to analyze its binding dynamics with specific antibodies:
- Binding Constants : The dissociation constant (Kd) and association constant (Ka) were determined using non-linear regression analysis .
- Experimental Setup : GST was bioconjugated with F5M under controlled conditions to ensure proper labeling and subsequent interaction studies.
Advantages and Limitations
Advantages:
- High specificity for thiol groups allows for targeted labeling.
- Fluorescent properties enable real-time monitoring of biological processes.
- Versatile applications across various fields including microbiology, biochemistry, and molecular biology.
Limitations:
- Potential interference from other reactive species in complex biological systems.
- Requires careful optimization of labeling conditions to avoid protein denaturation.
Q & A
Basic Questions
Q. How does Fluorescein-5-maleimide selectively conjugate with thiol groups in biomolecules under physiological conditions?
this compound reacts with free thiol (-SH) groups via its maleimide moiety, forming stable thioether bonds at physiological pH (6.5–7.5). This selectivity arises from the maleimide group’s electrophilicity, which preferentially targets nucleophilic thiols over other residues (e.g., amines). For validation, perform SDS-PAGE with fluorescence detection to confirm conjugation, comparing labeled vs. unlabeled samples .
Q. What are the optimal pH and buffer conditions for efficient thiol labeling?
Use buffers like 50 mM sodium phosphate (pH 7.0) with 1 mM EDTA to maintain pH stability and prevent metal-induced oxidation of thiols. Avoid Tris-based buffers, as their primary amines may compete with thiols at higher pH (>8.0). Pre-reduce disulfide bonds with TCEP (1–5 mM) to maximize free thiol availability .
Q. What spectroscopic properties make this compound suitable for fluorescence microscopy?
The fluorescein moiety exhibits excitation/emission maxima at ~494 nm/519 nm (green spectrum). Its high quantum yield (~0.95) ensures bright signals in imaging. For live-cell tracking, use low-light settings to minimize photobleaching, and confirm specificity with unlabeled controls .
Q. What controls are necessary to validate successful conjugation?
Include:
- Negative control : Labeling in the absence of thiols (e.g., cysteine-free mutants).
- Competitive inhibition : Pre-treatment with excess thiol-blocking agents (e.g., N-ethylmaleimide).
- Fluorescence validation : Compare SDS-PAGE Coomassie staining (protein loading) with fluorescence imaging (labeling efficiency) .
Advanced Questions
Q. How can researchers resolve contradictions in labeling efficiency across cysteine mutants?
Solvent accessibility of cysteine residues critically impacts labeling. Use computational tools like PyMOL or MD simulations to predict surface-exposed cysteines. Experimentally, employ site-directed mutagenesis to engineer solvent-accessible cysteines, and validate via fluorescence quenching assays in denaturing vs. native conditions .
Q. What strategies minimize non-specific binding in complex biological systems?
- Optimize molar ratios : Use a 25-fold molar excess of this compound over target thiols to ensure saturation while avoiding aggregation.
- Quench unreacted dye : Post-labeling, add excess β-mercaptoethanol or cysteine to block residual maleimide groups.
- Purify conjugates : Size-exclusion chromatography removes unbound dye .
Q. How to design time-resolved experiments to track dynamic thiol-disulfide exchange?
Combine this compound with time-lapse microscopy. For example:
- Stimulate cells with redox agents (e.g., H₂O₂ or DTT) to induce disulfide shuffling.
- Image at 15-minute intervals using multi-well plates, ensuring minimal light exposure.
- Quantify fluorescence intensity changes in specific subcellular compartments (e.g., mitochondria) .
Q. How does solvent polarity influence fluorescence quantum yield?
Fluorescein’s quantum yield decreases in hydrophobic environments due to restricted molecular rotation. To assess, compare emission spectra of conjugates in aqueous vs. lipid-rich matrices (e.g., liposomes). Use Förster resonance energy transfer (FRET) with hydrophobic acceptors (e.g., Nile Red) to map microenvironments .
Q. What computational tools predict cysteine accessibility for labeling?
Tools like APBS (Adaptive Poisson-Boltzmann Solver) calculate electrostatic potentials to identify solvent-accessible cysteines. Pair with Rosetta for energy-based scoring of cysteine reactivity. Validate predictions via mass spectrometry after limited proteolysis .
Q. How to quantify labeling efficiency in spectrophotometry while avoiding pitfalls?
- Absorbance method : Measure absorbance at 494 nm (Fluorescein) and 280 nm (protein). Correct for dye contribution at 280 nm using the formula:
where and (protein-specific).
Propriétés
IUPAC Name |
1-(3',6'-dihydroxy-3-oxospiro[2-benzofuran-1,9'-xanthene]-5-yl)pyrrole-2,5-dione | |
---|---|---|
Source | PubChem | |
URL | https://pubchem.ncbi.nlm.nih.gov | |
Description | Data deposited in or computed by PubChem | |
InChI |
InChI=1S/C24H13NO7/c26-13-2-5-17-19(10-13)31-20-11-14(27)3-6-18(20)24(17)16-4-1-12(9-15(16)23(30)32-24)25-21(28)7-8-22(25)29/h1-11,26-27H | |
Source | PubChem | |
URL | https://pubchem.ncbi.nlm.nih.gov | |
Description | Data deposited in or computed by PubChem | |
InChI Key |
AYDAHOIUHVUJHQ-UHFFFAOYSA-N | |
Source | PubChem | |
URL | https://pubchem.ncbi.nlm.nih.gov | |
Description | Data deposited in or computed by PubChem | |
Canonical SMILES |
C1=CC2=C(C=C1N3C(=O)C=CC3=O)C(=O)OC24C5=C(C=C(C=C5)O)OC6=C4C=CC(=C6)O | |
Source | PubChem | |
URL | https://pubchem.ncbi.nlm.nih.gov | |
Description | Data deposited in or computed by PubChem | |
Molecular Formula |
C24H13NO7 | |
Source | PubChem | |
URL | https://pubchem.ncbi.nlm.nih.gov | |
Description | Data deposited in or computed by PubChem | |
DSSTOX Substance ID |
DTXSID80226327 | |
Record name | Fluorescein 5-maleimide | |
Source | EPA DSSTox | |
URL | https://comptox.epa.gov/dashboard/DTXSID80226327 | |
Description | DSSTox provides a high quality public chemistry resource for supporting improved predictive toxicology. | |
Molecular Weight |
427.4 g/mol | |
Source | PubChem | |
URL | https://pubchem.ncbi.nlm.nih.gov | |
Description | Data deposited in or computed by PubChem | |
CAS No. |
75350-46-8 | |
Record name | Fluorescein 5-maleimide | |
Source | ChemIDplus | |
URL | https://pubchem.ncbi.nlm.nih.gov/substance/?source=chemidplus&sourceid=0075350468 | |
Description | ChemIDplus is a free, web search system that provides access to the structure and nomenclature authority files used for the identification of chemical substances cited in National Library of Medicine (NLM) databases, including the TOXNET system. | |
Record name | Fluorescein 5-maleimide | |
Source | EPA DSSTox | |
URL | https://comptox.epa.gov/dashboard/DTXSID80226327 | |
Description | DSSTox provides a high quality public chemistry resource for supporting improved predictive toxicology. | |
Retrosynthesis Analysis
AI-Powered Synthesis Planning: Our tool employs the Template_relevance Pistachio, Template_relevance Bkms_metabolic, Template_relevance Pistachio_ringbreaker, Template_relevance Reaxys, Template_relevance Reaxys_biocatalysis model, leveraging a vast database of chemical reactions to predict feasible synthetic routes.
One-Step Synthesis Focus: Specifically designed for one-step synthesis, it provides concise and direct routes for your target compounds, streamlining the synthesis process.
Accurate Predictions: Utilizing the extensive PISTACHIO, BKMS_METABOLIC, PISTACHIO_RINGBREAKER, REAXYS, REAXYS_BIOCATALYSIS database, our tool offers high-accuracy predictions, reflecting the latest in chemical research and data.
Strategy Settings
Precursor scoring | Relevance Heuristic |
---|---|
Min. plausibility | 0.01 |
Model | Template_relevance |
Template Set | Pistachio/Bkms_metabolic/Pistachio_ringbreaker/Reaxys/Reaxys_biocatalysis |
Top-N result to add to graph | 6 |
Feasible Synthetic Routes
Avertissement et informations sur les produits de recherche in vitro
Veuillez noter que tous les articles et informations sur les produits présentés sur BenchChem sont destinés uniquement à des fins informatives. Les produits disponibles à l'achat sur BenchChem sont spécifiquement conçus pour des études in vitro, qui sont réalisées en dehors des organismes vivants. Les études in vitro, dérivées du terme latin "in verre", impliquent des expériences réalisées dans des environnements de laboratoire contrôlés à l'aide de cellules ou de tissus. Il est important de noter que ces produits ne sont pas classés comme médicaments et n'ont pas reçu l'approbation de la FDA pour la prévention, le traitement ou la guérison de toute condition médicale, affection ou maladie. Nous devons souligner que toute forme d'introduction corporelle de ces produits chez les humains ou les animaux est strictement interdite par la loi. Il est essentiel de respecter ces directives pour assurer la conformité aux normes légales et éthiques en matière de recherche et d'expérimentation.