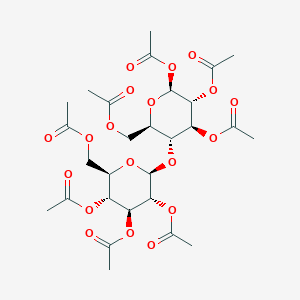
D-Cellobiose octaacetate
Vue d'ensemble
Description
D-Cellobiose octaacetate (CAS: 5346-90-7; alternative CAS: 3616-19-1) is a fully acetylated derivative of cellobiose, a disaccharide composed of two β-1,4-linked glucose units. Its molecular formula is C₂₈H₃₈O₁₉, with a molecular weight of 678.59 g/mol . Structural analysis reveals eight acetyl groups (-OAc) replacing hydroxyl groups on the glucose residues, enhancing its hydrophobicity and stability compared to native cellobiose.
Méthodes De Préparation
Historical Context: Braun’s Classical Synthesis of α-D-Cellobiose Octaacetate
The foundational method for α-D-cellobiose octaacetate synthesis, described by Braun in 1943, involves the acetylation of cellulose with acetic anhydride and sulfuric acid . In this process, 100g of cellulose is treated with 400mL acetic anhydride and 36mL sulfuric acid at 45–55°C, followed by a seven-day reaction period at 35°C . Crystallization begins spontaneously after two days, but the crude product requires extensive purification via methanol and chloroform recrystallization. Braun’s method yields 65–69g of product, albeit with significant impurities (52% purity pre-purification) .
Limitations of the Braun Method
The Braun synthesis suffers from two critical drawbacks:
-
Excessive Reaction Volume : Quenching the reaction mixture into 20L of water creates logistical challenges for large-scale production .
-
Prolonged Reaction Time : The seven-day incubation period delays throughput and increases energy costs .
Attempts to modify these parameters without process optimization led to inconsistent results, such as unfilterable slurries at elevated temperatures .
Modern Advances in α-D-Cellobiose Octaacetate Synthesis
The patent WO1993022322A1 introduces a streamlined one-step process that addresses Braun’s limitations through controlled reaction conditions and optimized work-up procedures .
Improved Acetylative Degradation of Cellulose
The refined method involves:
-
Reagent Ratios : For every 100g cellulose, 200–400g acetic anhydride, 50–200g acetic acid, and 10–70g sulfuric acid are used .
-
Temperature Control : Reactions proceed at 45–55°C for 14–20 hours, with seeding to initiate crystallization .
-
Alcohol Quench : Methanol (50–300% v/v) replaces water for acetic anhydride neutralization, reducing work-up volume .
Table 1: Comparison of Braun vs. Improved Synthesis
Parameter | Braun Method (1943) | Improved Method (WO1993022322A1) |
---|---|---|
Reaction Volume | 20L (quench) | 3L (methanol quench) |
Reaction Time | 7 days | 18–30 hours |
Yield (per 100g cellulose) | 65–69g | 284–788g |
Purity (HPLC) | 52% (crude) | 93–95% (crude) |
Work-up Solvent | Water | Methanol |
Role of Cellulose Acetate Feedstock
Substituting cellulose with partially acetylated cellulose acetate (e.g., CA-398-30, 2.45 acetyl groups per glucose unit) enhances yield but extends reaction time to 30 hours . This trade-off arises from the need for incremental deacetylation before chain scission.
Mechanistic Insights and Process Optimization
Acid-Catalyzed Depolymerization
Sulfuric acid catalyzes both acetylation and glycosidic bond cleavage. At 50°C, the balance between these competing reactions shifts toward controlled depolymerization, favoring cellobiose octaacetate over glucose pentaacetate or higher oligomers .
Seeding Strategies
Introducing α-D-cellobiose octaacetate seed crystals (8g per 544g feedstock) during the initial reaction phase accelerates nucleation, reducing induction periods from 48 hours to <5 hours .
Experimental Validation and Analytical Data
Synthesis from Cellulose
A representative experiment yields 788g product from 1044g cellulose, achieving 93% purity post-methanol wash . Key analytical data include:
Table 2: Physicochemical Properties of α-D-Cellobiose Octaacetate
Scalability and Reproducibility
Batch sizes up to 3L demonstrate consistent yields (75–85% theoretical), with no reported side products exceeding 7% by HPLC .
Industrial and Pharmaceutical Implications
The improved process’s efficiency supports cost-effective production of α-D-cellobiose octaacetate for prodrug synthesis, such as tigogenin cellobioside (anti-hypercholesterolemia) . Reduced solvent volumes align with green chemistry principles, lowering waste disposal costs.
Analyse Des Réactions Chimiques
Types of Reactions: D-Cellobiose octaacetate undergoes various chemical reactions, including:
Hydrolysis: The compound can be hydrolyzed to yield cellobiose and acetic acid.
Reduction: Reduction reactions can convert the acetyl groups back to hydroxyl groups, regenerating cellobiose.
Substitution: The acetyl groups can be substituted with other functional groups through nucleophilic substitution reactions.
Common Reagents and Conditions:
Hydrolysis: Water or dilute acids/bases at elevated temperatures.
Reduction: Reducing agents such as lithium aluminum hydride (LiAlH4) or sodium borohydride (NaBH4).
Substitution: Nucleophiles such as amines or alcohols in the presence of a base.
Major Products:
Hydrolysis: Cellobiose and acetic acid.
Reduction: Cellobiose.
Substitution: Various substituted cellobiose derivatives depending on the nucleophile used
Applications De Recherche Scientifique
Glycobiology Research
D-Cellobiose octaacetate serves as a valuable tool in glycobiology for studying carbohydrate structures and interactions. It aids researchers in understanding complex biological processes involving glycosylation and carbohydrate recognition .
Drug Development
This compound is used in synthesizing glycosylated drugs, enhancing their efficacy and stability. Its ability to form stable complexes with various pharmaceuticals makes it essential in developing new medications .
Food Industry
In the food sector, this compound can be employed as a food additive to modify texture and stability, thus improving product quality. It helps in formulating products that require specific textural properties .
Biotechnology
This compound acts as a substrate for glycosyltransferases in enzyme research. This application allows scientists to explore enzyme mechanisms and develop biocatalysts that can be used in various biotechnological processes .
Analytical Chemistry
In analytical chemistry, this compound is utilized in chromatographic techniques for separating and analyzing carbohydrates. It provides precise insights into carbohydrate composition across different samples, which is crucial for quality control and research purposes .
Data Table: Applications Overview
Application Area | Description | Key Benefits |
---|---|---|
Glycobiology | Study of carbohydrate structures and interactions | Understanding biological processes |
Drug Development | Synthesis of glycosylated compounds | Enhanced drug efficacy and stability |
Food Industry | Food additive for modifying texture and stability | Improved product quality |
Biotechnology | Substrate for glycosyltransferases | Exploration of enzyme mechanisms |
Analytical Chemistry | Chromatographic separation and analysis of carbohydrates | Precise carbohydrate composition insights |
Case Study 1: Glycosylation in Drug Development
A study published in Journal of Medicinal Chemistry demonstrated the synthesis of a novel glycosylated drug using this compound as a precursor. The results indicated improved bioavailability compared to non-glycosylated counterparts, highlighting the compound's role in enhancing pharmaceutical properties .
Case Study 2: Enzyme Mechanism Exploration
Research conducted at a leading biotechnology lab utilized this compound to investigate the substrate specificity of various glycosyltransferases. The findings revealed critical insights into enzyme-substrate interactions that could lead to the development of more efficient biocatalysts for industrial applications .
Mécanisme D'action
The mechanism of action of D-Cellobiose octaacetate involves its interaction with specific molecular targets, primarily enzymes that recognize carbohydrate structures. The acetyl groups on the molecule can influence its binding affinity and specificity towards these enzymes. The pathways involved include:
Enzyme Inhibition: The compound can act as an inhibitor for certain glycosidases by mimicking the natural substrate and blocking the active site.
Drug Delivery: The acetylated form can enhance the stability and solubility of drugs, facilitating their delivery to target sites
Comparaison Avec Des Composés Similaires
Physical Properties :
- Purity : Commercially available samples typically exceed ≥98% purity .
- Applications : Key uses include chemical synthesis (e.g., as a precursor for glycosylation reactions ), induction of cellulases in microbial systems , and enhancement of ionic conductivity in solid polymer electrolytes for lithium batteries .
Structural Analogs: Disaccharide Octaacetates
The following table compares D-Cellobiose octaacetate with structurally related disaccharide octaacetates:
*Discrepancy in melting points for this compound may arise from polymorphic variations .
Functional Comparisons
Sucrose Octaacetate
- Physicochemical Properties : Exhibits a lower melting point (89–92°C) and higher solubility in organic solvents compared to this compound. Demonstrates HLB 7–8 , enabling its use in stable oil/water emulsions (54–60% dispersity) and foam formation .
- Antimicrobial Activity : Effective against fungi (e.g., Aspergillus oryzae) and yeasts (Candida albicans) at 1–10 mg/cm³ but inactive against Gram-positive/-negative bacteria .
- Regulatory Status : Approved by the U.S. FDA as a food additive and pesticide inert ingredient due to rapid biodegradation and low toxicity .
β-D-Maltose Octaacetate
- Industrial Use : Functions as a CO₂-philic surfactant for microemulsions in green chemistry applications .
This compound
- Unique Applications: Cellulase Induction: At 0.1% concentration, induces Penicillium janthinellum to produce 0.8 IU/mL filter paper activity and 4.7 IU/mL β-glucosidase, outperforming gentiobiose and trehalose derivatives . Battery Technology: Modifies Li⁺ coordination in poly(vinylidene fluoride-co-hexafluoropropylene) electrolytes, increasing room-temperature ionic conductivity by weakening Li⁺-polymer interactions .
Activité Biologique
D-Cellobiose octaacetate is a synthetic derivative of the disaccharide cellobiose, characterized by its full acetylation, which enhances its solubility in organic solvents and alters its reactivity. This compound has garnered interest in biochemical research due to its potential applications in enzyme studies, carbohydrate metabolism, and pharmaceutical synthesis.
- Molecular Formula : C₂₈H₃₈O₁₉
- Molecular Weight : 678.59 g/mol
- Melting Point : 224-231 °C
- Appearance : White solid
Synthesis
This compound is synthesized through the acetylation of cellobiose, where hydroxyl groups are replaced with acetyl groups. This process typically involves:
- Treating cellulose acetate with acetic anhydride and acetic acid.
- Heating the mixture to facilitate acetylation.
- Purifying the product through recrystallization.
This method yields high-purity this compound, suitable for various biological applications .
Enzyme Interaction
This compound serves primarily as a substrate for enzymes that hydrolyze β-1,4 glycosidic bonds, such as:
- Cellobiase
- β-Glucosidase
These enzymes are crucial for understanding carbohydrate metabolism and enzymatic kinetics. The compound can also act as an inhibitor for certain glycoside hydrolases, allowing researchers to explore enzyme mechanisms and interactions .
Table 1: Enzymatic Activity with this compound
Enzyme | Activity Type | Observations |
---|---|---|
Cellobiase | Substrate | Hydrolysis leads to glucose production |
β-Glucosidase | Substrate | Kinetic studies reveal enzyme efficiency |
Glycoside Hydrolases | Inhibitor | Competitive inhibition observed |
Applications in Research
This compound is utilized in various research fields:
- Carbohydrate Metabolism : It helps elucidate the roles of enzymes involved in carbohydrate digestion.
- Pharmaceutical Synthesis : The compound is a precursor for synthesizing β-cellobioside moieties used in drug development .
- Biofuels Research : Its structural properties allow for studies on cellulose interactions, contributing to biofuel production methods.
Case Study 1: Enzyme Kinetics
In a study examining the kinetics of cellobiase, researchers used this compound as a substrate. The results indicated a significant increase in enzyme activity compared to unmodified cellobiose, suggesting that the acetylation enhances substrate recognition and hydrolysis rates .
Case Study 2: Inhibition Studies
Another investigation focused on the inhibitory effects of this compound on glycoside hydrolases. The findings revealed that at specific concentrations, the compound could effectively inhibit enzyme activity, providing insights into potential therapeutic applications for metabolic disorders .
Q & A
Basic Research Questions
Q. What are the optimal synthetic routes for preparing high-purity D-Cellobiose octaacetate, and how is yield maximized?
- Methodology : Copper-catalyzed anomeric O-arylation is a robust method, where α-D-cellobiose octaacetate is reacted with benzylamine in THF/acetone under mild heating (50°C). Purification via flash chromatography (cyclohexane/EtOAc) ensures separation of anomeric mixtures (α:β = 1.3:1) . For large-scale synthesis, acetylation of cellobiose using acetic anhydride and a catalyst (e.g., H₂SO₄) followed by recrystallization from ethanol yields ≥98% purity .
- Key Metrics : Monitor reaction progress via TLC and confirm purity using HPLC (retention time: ~16.2 min for α-anomer) .
Q. Which analytical techniques are essential for structural confirmation and purity assessment?
- Nuclear Magnetic Resonance (NMR) : ¹H and ¹³C NMR resolve acetyl group positions and anomeric configuration. For example, α-D-cellobiose octaacetate shows distinct downfield shifts for anomeric protons (~6.2 ppm) .
- High-Performance Liquid Chromatography (HPLC) : Quantify purity using a C18 column with acetonitrile/water gradients. Purity ≥98% is achievable with retention times validated against standards .
- Infrared Spectroscopy (IR) : Confirm esterification via C=O stretches (~1740 cm⁻¹) and absence of hydroxyl peaks .
Q. How can researchers standardize protocols for assessing batch-to-batch consistency?
- Quality Control : Use HPLC with UV detection (210 nm) to compare retention times and peak areas across batches. Validate against certified reference materials (e.g., ≥98% purity by TLC) .
- Thermal Analysis : Differential scanning calorimetry (DSC) confirms melting point consistency (225–229°C) .
Advanced Research Questions
Q. What mechanistic role does this compound play in enhancing ionic conductivity in solid polymer electrolytes (SPEs)?
- Mechanism : The compound’s acetyl C=O groups compete with anions (e.g., TFSI⁻) to coordinate Li⁺ ions, weakening polymer-Li⁺ interactions and increasing carrier mobility. This reduces crystallinity in SPEs (e.g., PVDF-HFP), boosting ionic conductivity to ~10⁻⁴ S/cm at 25°C .
- Experimental Design : Electrochemical impedance spectroscopy (EIS) measures conductivity, while X-ray diffraction (XRD) confirms reduced crystallinity. Symmetric Li|SPE|Li cells validate stability during cycling .
Q. How do solvent interactions with ionic liquids (e.g., EmimAc) influence this compound’s solvation behavior?
- NMR Insights : In EmimAc, hydrogen bonding occurs between cellobiose hydroxyls and both the acetate anion (CH₃COO⁻) and imidazolium cation (H2 proton). Acetylation eliminates these interactions, confirming hydrogen bonding as the primary dissolution driver .
- Variable-Temperature NMR : Track chemical shift changes to map solvation dynamics. Stoichiometric ratios (EmimAc:hydroxyl ≈ 3:4) indicate cooperative binding .
Q. How can contradictory structural data from NMR and XRD be resolved in polymorph studies?
- Multi-Technique Approach : Combine 2D NMR (e.g., HSQC, HMBC) to assign acetyl group positions with single-crystal XRD for absolute configuration. Discrepancies may arise from dynamic exchange in solution vs. static solid-state structures .
- Case Study : Conflicting anomeric ratios (α:β) in synthesis can be resolved by optimizing reaction conditions (e.g., solvent polarity, temperature) to favor kinetic vs. thermodynamic products .
Q. What environmental fate considerations are critical for lab-scale use of this compound?
- Biodegradation : Rapid microbial degradation (hours to days) occurs via esterase-mediated hydrolysis, releasing acetic acid and cellobiose. Monitor using OECD 301F biodegradation assays .
- Toxicity Mitigation : While acute toxicity is low (LD₅₀ > 2000 mg/kg in rats), implement waste neutralization protocols (e.g., alkaline hydrolysis) to prevent acetic acid accumulation .
Propriétés
IUPAC Name |
[(2R,3R,4S,5R,6S)-4,5,6-triacetyloxy-3-[(2S,3R,4S,5R,6R)-3,4,5-triacetyloxy-6-(acetyloxymethyl)oxan-2-yl]oxyoxan-2-yl]methyl acetate | |
---|---|---|
Source | PubChem | |
URL | https://pubchem.ncbi.nlm.nih.gov | |
Description | Data deposited in or computed by PubChem | |
InChI |
InChI=1S/C28H38O19/c1-11(29)37-9-19-21(39-13(3)31)23(40-14(4)32)26(43-17(7)35)28(46-19)47-22-20(10-38-12(2)30)45-27(44-18(8)36)25(42-16(6)34)24(22)41-15(5)33/h19-28H,9-10H2,1-8H3/t19-,20-,21-,22-,23+,24+,25-,26-,27-,28+/m1/s1 | |
Source | PubChem | |
URL | https://pubchem.ncbi.nlm.nih.gov | |
Description | Data deposited in or computed by PubChem | |
InChI Key |
WOTQVEKSRLZRSX-HYSGBLIFSA-N | |
Source | PubChem | |
URL | https://pubchem.ncbi.nlm.nih.gov | |
Description | Data deposited in or computed by PubChem | |
Canonical SMILES |
CC(=O)OCC1C(C(C(C(O1)OC(=O)C)OC(=O)C)OC(=O)C)OC2C(C(C(C(O2)COC(=O)C)OC(=O)C)OC(=O)C)OC(=O)C | |
Source | PubChem | |
URL | https://pubchem.ncbi.nlm.nih.gov | |
Description | Data deposited in or computed by PubChem | |
Isomeric SMILES |
CC(=O)OC[C@@H]1[C@H]([C@@H]([C@H]([C@@H](O1)OC(=O)C)OC(=O)C)OC(=O)C)O[C@H]2[C@@H]([C@H]([C@@H]([C@H](O2)COC(=O)C)OC(=O)C)OC(=O)C)OC(=O)C | |
Source | PubChem | |
URL | https://pubchem.ncbi.nlm.nih.gov | |
Description | Data deposited in or computed by PubChem | |
Molecular Formula |
C28H38O19 | |
Source | PubChem | |
URL | https://pubchem.ncbi.nlm.nih.gov | |
Description | Data deposited in or computed by PubChem | |
Molecular Weight |
678.6 g/mol | |
Source | PubChem | |
URL | https://pubchem.ncbi.nlm.nih.gov | |
Description | Data deposited in or computed by PubChem | |
CAS No. |
3616-19-1, 5346-90-7, 22352-19-8 | |
Record name | Cellobiose octaacetate | |
Source | ChemIDplus | |
URL | https://pubchem.ncbi.nlm.nih.gov/substance/?source=chemidplus&sourceid=0003616191 | |
Description | ChemIDplus is a free, web search system that provides access to the structure and nomenclature authority files used for the identification of chemical substances cited in National Library of Medicine (NLM) databases, including the TOXNET system. | |
Record name | D-Glucopyranose, 4-O-(2,3,4,6-tetra-O-acetyl-.beta.-D-glucopyranosyl)-, 1,2,3,6-tetraacetate | |
Source | EPA Chemicals under the TSCA | |
URL | https://www.epa.gov/chemicals-under-tsca | |
Description | EPA Chemicals under the Toxic Substances Control Act (TSCA) collection contains information on chemicals and their regulations under TSCA, including non-confidential content from the TSCA Chemical Substance Inventory and Chemical Data Reporting. | |
Record name | Alpha-D-cellobiose octaacetate | |
Source | European Chemicals Agency (ECHA) | |
URL | https://echa.europa.eu/substance-information/-/substanceinfo/100.023.912 | |
Description | The European Chemicals Agency (ECHA) is an agency of the European Union which is the driving force among regulatory authorities in implementing the EU's groundbreaking chemicals legislation for the benefit of human health and the environment as well as for innovation and competitiveness. | |
Explanation | Use of the information, documents and data from the ECHA website is subject to the terms and conditions of this Legal Notice, and subject to other binding limitations provided for under applicable law, the information, documents and data made available on the ECHA website may be reproduced, distributed and/or used, totally or in part, for non-commercial purposes provided that ECHA is acknowledged as the source: "Source: European Chemicals Agency, http://echa.europa.eu/". Such acknowledgement must be included in each copy of the material. ECHA permits and encourages organisations and individuals to create links to the ECHA website under the following cumulative conditions: Links can only be made to webpages that provide a link to the Legal Notice page. | |
Record name | Cellobiose octaacetate | |
Source | European Chemicals Agency (ECHA) | |
URL | https://echa.europa.eu/substance-information/-/substanceinfo/100.020.727 | |
Description | The European Chemicals Agency (ECHA) is an agency of the European Union which is the driving force among regulatory authorities in implementing the EU's groundbreaking chemicals legislation for the benefit of human health and the environment as well as for innovation and competitiveness. | |
Explanation | Use of the information, documents and data from the ECHA website is subject to the terms and conditions of this Legal Notice, and subject to other binding limitations provided for under applicable law, the information, documents and data made available on the ECHA website may be reproduced, distributed and/or used, totally or in part, for non-commercial purposes provided that ECHA is acknowledged as the source: "Source: European Chemicals Agency, http://echa.europa.eu/". Such acknowledgement must be included in each copy of the material. ECHA permits and encourages organisations and individuals to create links to the ECHA website under the following cumulative conditions: Links can only be made to webpages that provide a link to the Legal Notice page. | |
Record name | β-D-Glucopyranose, 4-O-(2,3,4,6-tetra-O-acetyl-α-D-glucopyranosyl)-, 1,2,3,6-tetraacetate | |
Source | European Chemicals Agency (ECHA) | |
URL | https://echa.europa.eu/information-on-chemicals | |
Description | The European Chemicals Agency (ECHA) is an agency of the European Union which is the driving force among regulatory authorities in implementing the EU's groundbreaking chemicals legislation for the benefit of human health and the environment as well as for innovation and competitiveness. | |
Explanation | Use of the information, documents and data from the ECHA website is subject to the terms and conditions of this Legal Notice, and subject to other binding limitations provided for under applicable law, the information, documents and data made available on the ECHA website may be reproduced, distributed and/or used, totally or in part, for non-commercial purposes provided that ECHA is acknowledged as the source: "Source: European Chemicals Agency, http://echa.europa.eu/". Such acknowledgement must be included in each copy of the material. ECHA permits and encourages organisations and individuals to create links to the ECHA website under the following cumulative conditions: Links can only be made to webpages that provide a link to the Legal Notice page. | |
Retrosynthesis Analysis
AI-Powered Synthesis Planning: Our tool employs the Template_relevance Pistachio, Template_relevance Bkms_metabolic, Template_relevance Pistachio_ringbreaker, Template_relevance Reaxys, Template_relevance Reaxys_biocatalysis model, leveraging a vast database of chemical reactions to predict feasible synthetic routes.
One-Step Synthesis Focus: Specifically designed for one-step synthesis, it provides concise and direct routes for your target compounds, streamlining the synthesis process.
Accurate Predictions: Utilizing the extensive PISTACHIO, BKMS_METABOLIC, PISTACHIO_RINGBREAKER, REAXYS, REAXYS_BIOCATALYSIS database, our tool offers high-accuracy predictions, reflecting the latest in chemical research and data.
Strategy Settings
Precursor scoring | Relevance Heuristic |
---|---|
Min. plausibility | 0.01 |
Model | Template_relevance |
Template Set | Pistachio/Bkms_metabolic/Pistachio_ringbreaker/Reaxys/Reaxys_biocatalysis |
Top-N result to add to graph | 6 |
Feasible Synthetic Routes
Avertissement et informations sur les produits de recherche in vitro
Veuillez noter que tous les articles et informations sur les produits présentés sur BenchChem sont destinés uniquement à des fins informatives. Les produits disponibles à l'achat sur BenchChem sont spécifiquement conçus pour des études in vitro, qui sont réalisées en dehors des organismes vivants. Les études in vitro, dérivées du terme latin "in verre", impliquent des expériences réalisées dans des environnements de laboratoire contrôlés à l'aide de cellules ou de tissus. Il est important de noter que ces produits ne sont pas classés comme médicaments et n'ont pas reçu l'approbation de la FDA pour la prévention, le traitement ou la guérison de toute condition médicale, affection ou maladie. Nous devons souligner que toute forme d'introduction corporelle de ces produits chez les humains ou les animaux est strictement interdite par la loi. Il est essentiel de respecter ces directives pour assurer la conformité aux normes légales et éthiques en matière de recherche et d'expérimentation.