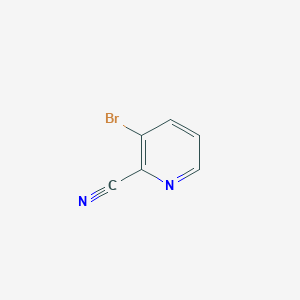
3-Bromo-2-cyanopyridine
Vue d'ensemble
Description
3-Bromo-2-cyanopyridine is an organic compound with the molecular formula C6H3BrN2 It is a derivative of pyridine, characterized by the presence of a bromine atom at the third position and a cyano group at the second position of the pyridine ring
Applications De Recherche Scientifique
3-Bromo-2-cyanopyridine has a wide range of applications in scientific research:
Mécanisme D'action
Target of Action
3-Bromo-2-cyanopyridine, also known as 3-Bromopyridine-2-carbonitrile , is a chemical compound that primarily targets the respiratory system . It is often used in the field of organic synthesis .
Mode of Action
It is known that cyanopyridines can react with thiol nucleophiles, such as those found in cysteine residues in proteins
Biochemical Pathways
This compound may be involved in the Suzuki–Miyaura cross-coupling reaction, a widely-applied transition metal-catalyzed carbon–carbon bond-forming reaction . This reaction involves the coupling of an organoboron compound (like this compound) with a halide or pseudohalide using a palladium catalyst .
Result of Action
Given its potential reactivity with thiol groups in cysteine residues, it could potentially alter protein structure and function . This could lead to a variety of downstream effects, depending on the specific proteins and pathways involved.
Action Environment
The action, efficacy, and stability of this compound can be influenced by various environmental factors. For instance, it is known to be moisture sensitive , suggesting that its stability and reactivity could be affected by humidity levels. Additionally, safety data suggests that it should be handled in a well-ventilated area , indicating that air quality and ventilation could impact its efficacy and safety.
Analyse Biochimique
Biochemical Properties
It is known that pyridine derivatives can interact with various enzymes and proteins, potentially influencing biochemical reactions
Molecular Mechanism
The molecular mechanism of action of 3-Bromo-2-cyanopyridine is not well-defined. It is known that pyridine derivatives can participate in various chemical reactions, potentially leading to changes in gene expression, enzyme inhibition or activation, and binding interactions with biomolecules . The specific molecular mechanisms associated with this compound have not been fully explored.
Méthodes De Préparation
Synthetic Routes and Reaction Conditions: 3-Bromo-2-cyanopyridine can be synthesized through several methods. One common approach involves the reaction of 3-bromo-2-pyridylcarboxaldehyde oxime with acetic anhydride. This reaction proceeds through the formation of an O-acetylated intermediate, which subsequently eliminates acetic acid to yield this compound .
Industrial Production Methods: Industrial production of this compound typically involves large-scale chemical synthesis using optimized reaction conditions to ensure high yield and purity. The specific details of industrial methods are often proprietary, but they generally follow similar principles to laboratory-scale synthesis, with adjustments for scalability and efficiency.
Analyse Des Réactions Chimiques
Types of Reactions: 3-Bromo-2-cyanopyridine undergoes various chemical reactions, including:
Substitution Reactions: It can participate in nucleophilic substitution reactions, where the bromine atom is replaced by other nucleophiles.
Coupling Reactions: It is commonly used in Suzuki–Miyaura coupling reactions, which involve the formation of carbon-carbon bonds between the pyridine ring and other aromatic or aliphatic groups.
Common Reagents and Conditions:
Suzuki–Miyaura Coupling: This reaction typically uses palladium catalysts, arylboronic acids, and bases such as potassium phosphate in solvents like 1,4-dioxane and water.
Nucleophilic Substitution: Common nucleophiles include amines, thiols, and alkoxides, often under basic conditions.
Major Products: The major products of these reactions depend on the specific reagents used. For example, Suzuki–Miyaura coupling with arylboronic acids produces biaryl compounds, while nucleophilic substitution with amines yields aminopyridine derivatives.
Comparaison Avec Des Composés Similaires
2-Cyanopyridine: Lacks the bromine atom, making it less reactive in certain substitution reactions.
3-Bromo-2-pyridinecarboxaldehyde: Contains an aldehyde group instead of a cyano group, leading to different reactivity and applications.
3-Bromo-4-cyanopyridine: The position of the cyano group affects its chemical properties and reactivity.
Uniqueness: 3-Bromo-2-cyanopyridine is unique due to the combination of the bromine and cyano groups, which confer distinct reactivity patterns and make it a versatile intermediate in organic synthesis. Its ability to participate in both nucleophilic substitution and coupling reactions broadens its utility in the synthesis of diverse chemical entities.
Activité Biologique
3-Bromo-2-cyanopyridine (CAS Number: 55758-02-6) is a heterocyclic compound that has garnered attention in medicinal chemistry due to its potential biological activities. This article explores the biological activity of this compound, focusing on its synthesis, cytotoxic effects, and potential applications in drug discovery.
- Molecular Formula : C6H3BrN2
- Molecular Weight : 183.01 g/mol
- Melting Point : 93-98 °C
Synthesis
The synthesis of this compound typically involves the reaction of 3-bromopyridine-N-oxide with trimethylsilyl cyanide in the presence of triethylamine under an inert atmosphere at elevated temperatures. The yield from this reaction can reach up to 89% under optimized conditions .
Anticancer Potential
Recent studies have highlighted the anticancer properties of derivatives of cyanopyridines, including this compound. A notable study synthesized various 3-cyanopyridine derivatives and evaluated their cytotoxicity against human cancer cell lines such as prostate carcinoma (PC-3), breast cancer (MDA-MB-231), and hepatocellular carcinoma (HepG2). The findings indicated that certain derivatives exhibited significant cytotoxic effects, with IC50 values indicating promising activity compared to standard chemotherapeutics like 5-fluorouracil (5-FU) .
Compound | Cell Line | IC50 (µM) |
---|---|---|
5e | PC-3 | 25 |
5e | MDA-MB-231 | 19 |
5e | HepG2 | Comparable to 5-FU |
4d | PC-3 | 53 |
4d | MDA-MB-231 | 30 |
4d | HepG2 | 66 |
The study also reported that the compound 5e , which has a methoxy substitution, showed twice the activity against the PC-3 cell line compared to 5-FU, indicating its potential as a lead compound for further development in cancer therapy .
The mechanism underlying the anticancer activity of these compounds appears to involve the modulation of survivin, an inhibitor of apoptosis protein often overexpressed in cancer cells. The tested compounds induced apoptosis and necrosis in cancer cells, with significant reductions in survivin expression observed through Western blot analysis. This suggests that these compounds may promote proteasome-dependent degradation of survivin, leading to enhanced apoptotic signaling .
Case Studies
- Cytotoxicity Evaluation : A study utilized the sulforhodamine B (SRB) assay to evaluate the cytotoxicity of synthesized derivatives against various cancer cell lines. The results demonstrated that certain derivatives had potent anti-proliferative effects, warranting further investigation into their structure-activity relationships (SAR) .
- Safety Assessment : The cytotoxicity of these compounds was also assessed on normal cell lines such as WI-38 to evaluate their safety profile. The results indicated that while some derivatives were effective against cancer cells, they displayed lower toxicity towards normal cells, highlighting their potential therapeutic index .
Propriétés
IUPAC Name |
3-bromopyridine-2-carbonitrile | |
---|---|---|
Source | PubChem | |
URL | https://pubchem.ncbi.nlm.nih.gov | |
Description | Data deposited in or computed by PubChem | |
InChI |
InChI=1S/C6H3BrN2/c7-5-2-1-3-9-6(5)4-8/h1-3H | |
Source | PubChem | |
URL | https://pubchem.ncbi.nlm.nih.gov | |
Description | Data deposited in or computed by PubChem | |
InChI Key |
HCOPIUVJCIZALB-UHFFFAOYSA-N | |
Source | PubChem | |
URL | https://pubchem.ncbi.nlm.nih.gov | |
Description | Data deposited in or computed by PubChem | |
Canonical SMILES |
C1=CC(=C(N=C1)C#N)Br | |
Source | PubChem | |
URL | https://pubchem.ncbi.nlm.nih.gov | |
Description | Data deposited in or computed by PubChem | |
Molecular Formula |
C6H3BrN2 | |
Source | PubChem | |
URL | https://pubchem.ncbi.nlm.nih.gov | |
Description | Data deposited in or computed by PubChem | |
DSSTOX Substance ID |
DTXSID70355837 | |
Record name | 3-Bromo-2-cyanopyridine | |
Source | EPA DSSTox | |
URL | https://comptox.epa.gov/dashboard/DTXSID70355837 | |
Description | DSSTox provides a high quality public chemistry resource for supporting improved predictive toxicology. | |
Molecular Weight |
183.01 g/mol | |
Source | PubChem | |
URL | https://pubchem.ncbi.nlm.nih.gov | |
Description | Data deposited in or computed by PubChem | |
CAS No. |
55758-02-6 | |
Record name | 3-Bromo-2-cyanopyridine | |
Source | EPA DSSTox | |
URL | https://comptox.epa.gov/dashboard/DTXSID70355837 | |
Description | DSSTox provides a high quality public chemistry resource for supporting improved predictive toxicology. | |
Record name | 3-Bromopyridine-2-carbonitrile | |
Source | European Chemicals Agency (ECHA) | |
URL | https://echa.europa.eu/information-on-chemicals | |
Description | The European Chemicals Agency (ECHA) is an agency of the European Union which is the driving force among regulatory authorities in implementing the EU's groundbreaking chemicals legislation for the benefit of human health and the environment as well as for innovation and competitiveness. | |
Explanation | Use of the information, documents and data from the ECHA website is subject to the terms and conditions of this Legal Notice, and subject to other binding limitations provided for under applicable law, the information, documents and data made available on the ECHA website may be reproduced, distributed and/or used, totally or in part, for non-commercial purposes provided that ECHA is acknowledged as the source: "Source: European Chemicals Agency, http://echa.europa.eu/". Such acknowledgement must be included in each copy of the material. ECHA permits and encourages organisations and individuals to create links to the ECHA website under the following cumulative conditions: Links can only be made to webpages that provide a link to the Legal Notice page. | |
Synthesis routes and methods I
Procedure details
Synthesis routes and methods II
Procedure details
Synthesis routes and methods III
Procedure details
Synthesis routes and methods IV
Procedure details
Retrosynthesis Analysis
AI-Powered Synthesis Planning: Our tool employs the Template_relevance Pistachio, Template_relevance Bkms_metabolic, Template_relevance Pistachio_ringbreaker, Template_relevance Reaxys, Template_relevance Reaxys_biocatalysis model, leveraging a vast database of chemical reactions to predict feasible synthetic routes.
One-Step Synthesis Focus: Specifically designed for one-step synthesis, it provides concise and direct routes for your target compounds, streamlining the synthesis process.
Accurate Predictions: Utilizing the extensive PISTACHIO, BKMS_METABOLIC, PISTACHIO_RINGBREAKER, REAXYS, REAXYS_BIOCATALYSIS database, our tool offers high-accuracy predictions, reflecting the latest in chemical research and data.
Strategy Settings
Precursor scoring | Relevance Heuristic |
---|---|
Min. plausibility | 0.01 |
Model | Template_relevance |
Template Set | Pistachio/Bkms_metabolic/Pistachio_ringbreaker/Reaxys/Reaxys_biocatalysis |
Top-N result to add to graph | 6 |
Feasible Synthetic Routes
Avertissement et informations sur les produits de recherche in vitro
Veuillez noter que tous les articles et informations sur les produits présentés sur BenchChem sont destinés uniquement à des fins informatives. Les produits disponibles à l'achat sur BenchChem sont spécifiquement conçus pour des études in vitro, qui sont réalisées en dehors des organismes vivants. Les études in vitro, dérivées du terme latin "in verre", impliquent des expériences réalisées dans des environnements de laboratoire contrôlés à l'aide de cellules ou de tissus. Il est important de noter que ces produits ne sont pas classés comme médicaments et n'ont pas reçu l'approbation de la FDA pour la prévention, le traitement ou la guérison de toute condition médicale, affection ou maladie. Nous devons souligner que toute forme d'introduction corporelle de ces produits chez les humains ou les animaux est strictement interdite par la loi. Il est essentiel de respecter ces directives pour assurer la conformité aux normes légales et éthiques en matière de recherche et d'expérimentation.