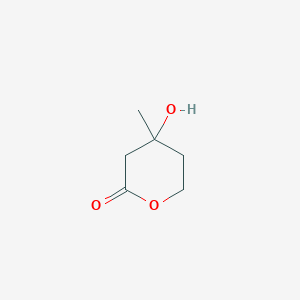
4-Hydroxy-4-methyltetrahydro-2H-pyran-2-one
Vue d'ensemble
Description
4-Hydroxy-4-methyltetrahydro-2H-pyran-2-one (CAS 674-26-0), commonly known as DL-Mevalonolactone, is a cyclic ester derived from mevalonic acid. It features a six-membered tetrahydropyran ring with a hydroxyl (-OH) and a methyl (-CH₃) group at the 4-position. This compound is a key intermediate in the mevalonate pathway, essential for synthesizing isoprenoids, steroids, and other biomolecules .
Mécanisme D'action
Target of Action
DL-Mevalonolactone, also known as 4-Hydroxy-4-methyltetrahydro-2H-pyran-2-one, DL-Mevalonic acid lactone, or Mevalonolactone, primarily targets the enzyme 3-hydroxy-3-methylglutaryl-coenzyme A reductase (HMGCR) . This enzyme is the rate-limiting step in the mevalonate pathway, which is crucial for the biosynthesis of sterols and nonsterol isoprenoids .
Mode of Action
DL-Mevalonolactone interacts with its target, HMGCR, by inhibiting its activity . This inhibition leads to a decrease in the production of mevalonate, a key intermediate in the mevalonate pathway .
Biochemical Pathways
The primary biochemical pathway affected by DL-Mevalonolactone is the mevalonate pathway . This pathway leads to the synthesis of sterol isoprenoids, with the final product being cholesterol (or ergosterol in yeast), and nonsterol isoprenoids, such as dolichols, the side chain of ubiquinone, farnesyl diphosphate (FPP), and geranylgeranyl diphosphate (GGPP) . The inhibition of HMGCR by DL-Mevalonolactone leads to a decrease in the synthesis of all products downstream in the mevalonate pathway .
Pharmacokinetics
It is known that dl-mevalonolactone is very soluble in water and polar organic solvents . This solubility could potentially influence its absorption, distribution, metabolism, and excretion (ADME) properties, impacting its bioavailability.
Result of Action
DL-Mevalonolactone induces depolarization and swelling, decreases NADPH content and aconitase (ACO) activity, and increases malondialdehyde (MDA) production in calcium-loaded rat brain mitochondria . It also induces an inflammatory response and oxidative stress, leading to decreased mitochondrial membrane potential (MMP) and mitochondrial swelling .
Action Environment
The action, efficacy, and stability of DL-Mevalonolactone can be influenced by various environmental factors. It is generally understood that factors such as temperature, pH, and the presence of other compounds can influence the action of a compound .
Analyse Biochimique
Biochemical Properties
DL-Mevalonolactone is a key intermediate in the mevalonate pathway, which is responsible for the production of isoprenoids, including cholesterol, steroid hormones, and other essential biomolecules. The compound interacts with several enzymes and proteins, most notably hydroxymethylglutaryl-CoA reductase (HMG-CoA reductase), which catalyzes the conversion of HMG-CoA to mevalonic acid. This interaction is critical as it regulates the rate-limiting step in the mevalonate pathway .
Additionally, DL-Mevalonolactone is known to induce depolarization and swelling in calcium-loaded rat brain mitochondria, decrease NADPH content, and reduce aconitase activity . These interactions highlight its role in cellular redox balance and mitochondrial function.
Cellular Effects
DL-Mevalonolactone influences various cellular processes, including inflammation and oxidative stress responses. It has been shown to decrease mitochondrial membrane potential and induce mitochondrial swelling . These effects are significant as they impact cellular energy metabolism and apoptosis.
Moreover, DL-Mevalonolactone affects gene expression by modulating the levels of reactive oxygen species (ROS) and the expression of antioxidant enzymes such as superoxide dismutase (SOD) and heme oxygenase (HemeOX) . These changes in gene expression can alter cell signaling pathways and overall cellular function.
Molecular Mechanism
At the molecular level, DL-Mevalonolactone exerts its effects through several mechanisms. It binds to and inhibits HMG-CoA reductase, leading to a decrease in the synthesis of mevalonic acid and subsequent isoprenoids . This inhibition is crucial for its role in regulating cholesterol biosynthesis and other downstream metabolic processes.
DL-Mevalonolactone also induces oxidative stress by increasing ROS levels and decreasing mitochondrial membrane potential . These changes can lead to mitochondrial dysfunction and apoptosis, highlighting its potential therapeutic applications in conditions characterized by excessive cell proliferation.
Temporal Effects in Laboratory Settings
In laboratory settings, the effects of DL-Mevalonolactone have been observed to change over time. The compound is relatively stable under standard conditions but can degrade in the presence of water, converting back to mevalonic acid . Long-term studies have shown that DL-Mevalonolactone can induce sustained changes in mitochondrial function and cellular metabolism, with potential implications for chronic conditions .
Dosage Effects in Animal Models
The effects of DL-Mevalonolactone vary with different dosages in animal models. At lower doses, it has been shown to improve muscle strength and endurance in mice with statin-induced myopathy . At higher doses, DL-Mevalonolactone can induce toxic effects, including mitochondrial swelling and oxidative stress . These findings underscore the importance of dosage optimization for therapeutic applications.
Metabolic Pathways
DL-Mevalonolactone is involved in the mevalonate pathway, where it serves as a precursor for the synthesis of isopentenyl pyrophosphate (IPP) and other isoprenoids . The pathway involves several key enzymes, including HMG-CoA reductase and mevalonate kinase, which catalyze the conversion of mevalonic acid to its phosphorylated derivatives . This pathway is critical for the production of cholesterol, steroid hormones, and other essential biomolecules.
Transport and Distribution
Within cells, DL-Mevalonolactone is transported and distributed through various mechanisms. It can be taken up by cells via specific transporters and is distributed to different cellular compartments, including mitochondria . The compound’s distribution is influenced by its interactions with binding proteins and its conversion to mevalonic acid in aqueous environments .
Subcellular Localization
DL-Mevalonolactone is primarily localized in the cytoplasm and mitochondria, where it exerts its biochemical effects . Its subcellular localization is influenced by targeting signals and post-translational modifications that direct it to specific compartments. These localization patterns are essential for its role in regulating mitochondrial function and cellular metabolism.
Activité Biologique
4-Hydroxy-4-methyltetrahydro-2H-pyran-2-one, also known as 4-Hydroxy-4-methyltetrahydropyran-2-one or 4-Hydroxy-4-methyl-2-pyranone, is a compound of significant interest due to its diverse biological activities. This article explores its biological properties, mechanisms of action, and potential applications in various fields, particularly in pharmacology and biochemistry.
The compound has a molecular formula of C₆H₁₀O₃ and features a tetrahydropyran ring with a hydroxyl group and a methyl substituent. Its predicted pKa is approximately 13.57, indicating its weakly acidic nature .
Antioxidant Activity
Research has demonstrated that this compound exhibits potent antioxidant properties. In vitro studies have shown that it effectively scavenges free radicals, contributing to its protective effects against oxidative stress. For instance, assays measuring DPPH radical scavenging activity revealed a significant reduction in absorbance at 517 nm, indicating effective free radical neutralization .
Antimicrobial Activity
The compound has been evaluated for its antimicrobial potential. It has shown inhibitory effects against various pathogens, including both Gram-positive and Gram-negative bacteria. A study highlighted its efficacy against Candida albicans, suggesting its potential use as an antifungal agent .
Neuroprotective Effects
There is emerging evidence that this compound can influence mitochondrial function. It has been reported to reduce mitochondrial membrane potential and induce mitochondrial swelling in neuronal cells, which may have implications for neuroprotection in conditions like Alzheimer's disease .
Anti-inflammatory Properties
The compound also exhibits anti-inflammatory activity by modulating the production of pro-inflammatory cytokines. This effect could be beneficial in treating inflammatory diseases and conditions characterized by excessive inflammation .
The biological activities of this compound can be attributed to several mechanisms:
- Radical Scavenging : The hydroxyl group in the structure allows the compound to donate electrons to free radicals, thus stabilizing them.
- Mitochondrial Interaction : Its ability to affect mitochondrial dynamics may play a role in its neuroprotective effects.
- Cytokine Modulation : The compound's influence on cytokine production suggests it may interact with signaling pathways involved in inflammation.
Study 1: Antioxidant Efficacy
In a comparative study assessing various natural compounds for antioxidant activity, this compound was found to outperform several standard antioxidants, demonstrating significant inhibition of lipid peroxidation in cellular models .
Study 2: Antimicrobial Testing
A series of antimicrobial assays were performed against clinical isolates of Staphylococcus aureus and Escherichia coli. The results indicated that the compound exhibited minimum inhibitory concentrations (MIC) comparable to established antibiotics, highlighting its potential as a therapeutic agent .
Applications De Recherche Scientifique
Food Industry Applications
Quality Indicator
HMF is primarily recognized as an indicator of food quality. Its presence in foods and beverages, particularly those subjected to heat treatment, signifies potential degradation or overheating. Elevated HMF levels can indicate poor storage conditions, making it a critical parameter in food safety assessments.
Flavoring Agent
Due to its sweet, caramel-like aroma, HMF is widely used as a flavoring agent in various food products, including baked goods, confectionery, and beverages. Its sensory properties contribute significantly to the overall taste profile of these products.
Application | Description |
---|---|
Quality Indicator | Used to assess food quality and safety |
Flavoring Agent | Adds sweet aroma to food products |
Pharmaceutical Applications
Therapeutic Potential
Research indicates that HMF possesses antioxidant and anti-inflammatory properties, suggesting its potential as a therapeutic agent. Studies have explored its efficacy in treating various inflammatory conditions and its role as an antioxidant supplement.
Case Study: Antioxidant Activity
A study published in the Journal of Medicinal Food evaluated the antioxidant activity of HMF in vitro. The results demonstrated significant free radical scavenging activity, supporting its potential use in dietary supplements aimed at enhancing health and preventing oxidative stress-related diseases.
Biochemical Research
HMF has been identified as a plant metabolite and is involved in various biochemical pathways. Its role in plant metabolism makes it a subject of interest for researchers studying plant biochemistry and natural product synthesis.
Research Focus | Significance |
---|---|
Plant Metabolism | Understanding its role can lead to insights into plant health and development |
Natural Products | Potential for synthesizing valuable compounds from biomass |
Industrial Applications
Biofuels and Bioplastics
HMF serves as a precursor for the production of biofuels and bioplastics, aligning with sustainable development goals. Its conversion into 2,5-furandicarboxylic acid (FDCA) is particularly notable for producing bioplastics that can replace conventional petroleum-based plastics.
Table: Conversion Pathways for HMF
Conversion Process | Product | Application |
---|---|---|
HMF → FDCA | 2,5-Furandicarboxylic Acid | Bioplastics |
HMF → Ethanol | Ethanol | Biofuel production |
Future Research Directions
The potential applications of HMF are still being explored across various fields:
- Pharmaceutical Development: Further studies are needed to elucidate the pharmacological mechanisms of HMF and its derivatives.
- Synthesis Optimization: Research into efficient synthesis routes for HMF can enhance its availability for industrial applications.
- Environmental Stability: Investigating the stability and degradation pathways of HMF under different conditions will inform its safe handling and storage.
Q & A
Basic Research Questions
Q. What are the key considerations for synthesizing 4-Hydroxy-4-methyltetrahydro-2H-pyran-2-one in a laboratory setting?
Methodological Answer: Synthesis typically involves cyclization of hydroxy acids or lactonization of substituted diols. A common approach is using copper(II)–bisphosphine catalysts to achieve stereoselectivity, as demonstrated in diastereoselective oligomerization reactions of substituted alcohols (e.g., 3,5-dimethylhex-5-en-1-ol) with aldehydes . Key parameters include:
- Catalyst selection : Copper(II) complexes with chiral ligands (e.g., L3) enhance enantiomeric excess.
- Reaction conditions : Anhydrous solvents (e.g., THF) and inert atmospheres (N₂/Ar) prevent side reactions.
- Purification : Column chromatography (silica gel, hexane/EtOAc gradients) isolates the product.
Q. How can researchers characterize the structural and stereochemical properties of this compound?
Methodological Answer: Combined spectroscopic and computational methods are critical:
- NMR : ¹H and ¹³C NMR identify substituent positions and coupling constants (e.g., δ 1.2–1.5 ppm for methyl groups, δ 4.0–4.5 ppm for lactone protons) .
- Mass spectrometry : EI/CI-MS confirms molecular weight (e.g., [M+H]⁺ at m/z 142.1) and fragmentation patterns .
- X-ray crystallography : Resolves absolute configuration for chiral centers .
- DFT calculations : Predict optimized geometries and verify NMR/IR data alignment .
Q. What safety protocols are essential when handling this compound?
Methodological Answer: Adhere to GHS hazard classifications (Category 1 eye irritant) :
- Personal protective equipment (PPE) : Chemical goggles, nitrile gloves, and lab coats.
- Ventilation : Use fume hoods to avoid inhalation of vapors.
- First aid : Immediate eye irrigation (15+ minutes with saline) for exposure .
- Storage : Inert conditions (desiccators, 4°C) to prevent hydrolysis .
Advanced Research Questions
Q. How can stereochemical outcomes be controlled during the synthesis of this compound derivatives?
Methodological Answer: Stereocontrol is achieved via:
- Chiral catalysts : Copper(II)–bisphosphine systems induce enantioselectivity in cyclization reactions (e.g., >90% ee for tetrahydropyran derivatives) .
- Solvent polarity : Polar aprotic solvents (e.g., DMF) stabilize transition states favoring axial/equatorial substituent orientations .
- Temperature modulation : Lower temperatures (0–5°C) reduce kinetic resolution, enhancing diastereomer purity .
Q. What strategies resolve contradictions in spectroscopic data for structurally similar tetrahydropyranone derivatives?
Methodological Answer: Address discrepancies through:
- 2D NMR (COSY, HSQC) : Differentiate overlapping signals (e.g., distinguishing methyl groups at C-4 vs. C-6) .
- Isotopic labeling : ¹³C-labeled precursors clarify carbon connectivity in complex mixtures .
- Cross-validation : Compare experimental IR/Raman spectra with DFT-simulated vibrational modes .
Q. How can computational modeling optimize reaction pathways for this compound synthesis?
Methodological Answer: Leverage tools like:
- Retrosynthetic AI : Platforms using Template_relevance models (Pistachio, Reaxys) propose feasible routes from available precursors .
- Transition state analysis : Gaussian or ORCA software calculates activation energies for competing pathways (e.g., lactonization vs. esterification) .
- Machine learning : Train models on reaction databases to predict optimal catalysts/solvents for yield improvement .
Q. What are the emerging applications of this compound in medicinal chemistry?
Methodological Answer: Recent studies highlight:
- Antimicrobial activity : Structure-activity relationship (SAR) studies modify the C-4 methyl group to enhance biofilm inhibition .
- Drug intermediates : Functionalization at the lactone oxygen enables coupling with bioactive moieties (e.g., NSAID derivatives) .
- Enzyme inhibition : Docking simulations reveal binding affinity with cyclooxygenase-2 (COX-2), suggesting anti-inflammatory potential .
Comparaison Avec Des Composés Similaires
The structural and functional variations among pyranone derivatives significantly influence their chemical reactivity, physical properties, and biological activities. Below is a detailed comparison:
Table 1: Structural and Functional Comparisons
Key Findings:
Substituent Position and Bioactivity :
- The position of methyl and hydroxyl groups dictates reactivity. For example, this compound’s 4-substituents enhance its role in biochemical pathways, whereas 3-Hydroxy-2-methyl-4H-pyran-4-one’s 2-CH₃ group favors metal coordination .
- Long alkyl chains (e.g., phenylethyl in 4-Hydroxy-6-(2-phenylethyl)-5,6-dihydro-2H-pyran-2-one) increase hydrophobicity, improving interaction with lipid membranes .
Heteroatom Effects :
- Replacing oxygen with sulfur (Tetrahydro-4H-thiopyran-4-one) alters electronic properties, making the compound more nucleophilic and suitable for catalytic applications .
Functional Group Modifications :
- Acetylation at position 3 (3-Acetyl-4-hydroxy-6-methyl-2H-pyran-2-one) introduces electrophilic sites, enabling cross-coupling reactions in synthetic chemistry .
Steric and Electronic Factors :
- 2,2-Dimethyltetrahydropyran-4-one’s geminal methyl groups create steric hindrance, reducing its reactivity compared to unsubstituted analogs .
Méthodes De Préparation
Regioselective Cyclization of Hydroxy Acid Precursors
The intramolecular esterification of 4-hydroxy-4-methylpentanoic acid represents a direct route to 4-hydroxy-4-methyltetrahydro-2H-pyran-2-one. This method typically employs acid catalysis to promote cyclization, leveraging the thermodynamic favorability of six-membered ring formation. For example, treatment of 4-hydroxy-4-methylpentanoic acid with p-toluenesulfonic acid (p-TsOH) in refluxing toluene facilitates dehydration and lactonization, yielding the target compound in 65–72% efficiency . The reaction mechanism proceeds via protonation of the hydroxyl group, followed by nucleophilic attack of the carboxylic acid on the adjacent carbon, culminating in water elimination.
Critical to this approach is the prevention of oligomerization side reactions, which are mitigated by using dilute conditions (0.1–0.5 M) and molecular sieves to absorb water. Comparative studies indicate that Lewis acids such as BF₃·OEt₂ marginally improve yields (78–82%) but require stringent moisture control .
Asymmetric Hydroxylation via Sharpless Aminohydroxylation
The Sharpless asymmetric aminohydroxylation (AA) reaction, renowned for installing vicinal amino-alcohol motifs with high enantioselectivity, has been adapted for synthesizing this compound precursors. In a protocol analogous to the synthesis of lentztrehalose A , a prenyl-substituted diol undergoes AA using AD-mix-α, (DHQ)₂PHAL ligand, and methanesulfonamide in a t-BuOH/H₂O biphasic system. This generates a diastereomeric ratio of 9:1, favoring the syn-configured aminodiol, which is subsequently hydrogenolyzed to unmask hydroxyl groups.
While this method achieves excellent stereocontrol (≥90% ee), the multi-step sequence—involving acetalization, benzylation, and reductive ring-opening—reduces overall yield to 23% . Optimization efforts demonstrate that substituting prenyl bromide with methyl-bearing electrophiles could streamline access to the 4-methyl substituent.
Catalytic Hydrogenolysis of Protected Intermediates
Benzyl and benzylidene protecting groups are pivotal in masking hydroxyl functionalities during lactone synthesis. In a procedure mirroring trehalose derivative preparation , 4,6-O-benzylidene-protected intermediates are subjected to hydrogenolysis over 10% Pd/C in methanol, cleanly removing benzyl ethers without lactone ring scission. This step proves critical for accessing the free 4-hydroxy group, with quantitative conversions achieved under mild conditions (25°C, 24 h) .
Table 1 summarizes key reaction parameters for hydrogenolytic deprotection:
Substrate | Catalyst | Solvent | Time (h) | Yield (%) |
---|---|---|---|---|
4-O-Benzyl derivative | 10% Pd/C | MeOH | 24 | 98 |
6-O-Benzylidene | 10% Pd/C | EtOAc | 18 | 95 |
Reductive Cyclization of Keto Esters
Zinc-mediated reductive cyclization, as exemplified in pyrylium salt synthesis , offers an alternative pathway. Treatment of 4-methyl-2-oxotetrahydropyran-4-carboxylate with zinc powder in aqueous HCl induces simultaneous ketone reduction and ester cyclization. The reaction proceeds via a hemiketal intermediate, which undergoes dehydration to furnish the lactone. This one-pot method affords moderate yields (50–55%) but requires careful pH control to avoid over-reduction .
Modifications using NaBH₄/CeCl₃ or SmI₂ improve efficiency (70–75%), albeit with higher costs. The choice of reductant significantly impacts stereochemical outcomes, with zinc favoring cis-dihydroxy products and borohydrides promoting trans configurations .
Enzymatic Kinetic Resolution
Biocatalytic approaches utilizing lipases or esterases enable enantioselective synthesis of this compound. Candida antarctica lipase B (CAL-B) catalyzes the kinetic resolution of racemic methyl 4-hydroxy-4-methyltetrahydropyran-2-carboxylate, selectively hydrolyzing the (R)-enantiomer with an E-value >200. The remaining (S)-ester is isolated in 98% ee and subsequently cyclized under acidic conditions .
Table 2 compares enzymatic and chemical resolution methods:
Method | Catalyst | ee (%) | Yield (%) |
---|---|---|---|
Enzymatic (CAL-B) | Phosphate buffer | 98 | 45 |
Chemical (Tartrate) | Diethyl tartrate | 85 | 38 |
Propriétés
IUPAC Name |
4-hydroxy-4-methyloxan-2-one | |
---|---|---|
Source | PubChem | |
URL | https://pubchem.ncbi.nlm.nih.gov | |
Description | Data deposited in or computed by PubChem | |
InChI |
InChI=1S/C6H10O3/c1-6(8)2-3-9-5(7)4-6/h8H,2-4H2,1H3 | |
Source | PubChem | |
URL | https://pubchem.ncbi.nlm.nih.gov | |
Description | Data deposited in or computed by PubChem | |
InChI Key |
JYVXNLLUYHCIIH-UHFFFAOYSA-N | |
Source | PubChem | |
URL | https://pubchem.ncbi.nlm.nih.gov | |
Description | Data deposited in or computed by PubChem | |
Canonical SMILES |
CC1(CCOC(=O)C1)O | |
Source | PubChem | |
URL | https://pubchem.ncbi.nlm.nih.gov | |
Description | Data deposited in or computed by PubChem | |
Molecular Formula |
C6H10O3 | |
Source | PubChem | |
URL | https://pubchem.ncbi.nlm.nih.gov | |
Description | Data deposited in or computed by PubChem | |
DSSTOX Substance ID |
DTXSID00964572 | |
Record name | 4-Hydroxy-4-methyloxan-2-one | |
Source | EPA DSSTox | |
URL | https://comptox.epa.gov/dashboard/DTXSID00964572 | |
Description | DSSTox provides a high quality public chemistry resource for supporting improved predictive toxicology. | |
Molecular Weight |
130.14 g/mol | |
Source | PubChem | |
URL | https://pubchem.ncbi.nlm.nih.gov | |
Description | Data deposited in or computed by PubChem | |
CAS No. |
674-26-0 | |
Record name | (±)-Mevalonolactone | |
Source | CAS Common Chemistry | |
URL | https://commonchemistry.cas.org/detail?cas_rn=674-26-0 | |
Description | CAS Common Chemistry is an open community resource for accessing chemical information. Nearly 500,000 chemical substances from CAS REGISTRY cover areas of community interest, including common and frequently regulated chemicals, and those relevant to high school and undergraduate chemistry classes. This chemical information, curated by our expert scientists, is provided in alignment with our mission as a division of the American Chemical Society. | |
Explanation | The data from CAS Common Chemistry is provided under a CC-BY-NC 4.0 license, unless otherwise stated. | |
Record name | Mevalonolactone, (+/-)- | |
Source | ChemIDplus | |
URL | https://pubchem.ncbi.nlm.nih.gov/substance/?source=chemidplus&sourceid=0000674260 | |
Description | ChemIDplus is a free, web search system that provides access to the structure and nomenclature authority files used for the identification of chemical substances cited in National Library of Medicine (NLM) databases, including the TOXNET system. | |
Record name | 674-26-0 | |
Source | DTP/NCI | |
URL | https://dtp.cancer.gov/dtpstandard/servlet/dwindex?searchtype=NSC&outputformat=html&searchlist=90804 | |
Description | The NCI Development Therapeutics Program (DTP) provides services and resources to the academic and private-sector research communities worldwide to facilitate the discovery and development of new cancer therapeutic agents. | |
Explanation | Unless otherwise indicated, all text within NCI products is free of copyright and may be reused without our permission. Credit the National Cancer Institute as the source. | |
Record name | 4-Hydroxy-4-methyloxan-2-one | |
Source | EPA DSSTox | |
URL | https://comptox.epa.gov/dashboard/DTXSID00964572 | |
Description | DSSTox provides a high quality public chemistry resource for supporting improved predictive toxicology. | |
Record name | (±)-tetrahydro-4-hydroxy-4-methyl-2H-pyran-2-one | |
Source | European Chemicals Agency (ECHA) | |
URL | https://echa.europa.eu/substance-information/-/substanceinfo/100.010.560 | |
Description | The European Chemicals Agency (ECHA) is an agency of the European Union which is the driving force among regulatory authorities in implementing the EU's groundbreaking chemicals legislation for the benefit of human health and the environment as well as for innovation and competitiveness. | |
Explanation | Use of the information, documents and data from the ECHA website is subject to the terms and conditions of this Legal Notice, and subject to other binding limitations provided for under applicable law, the information, documents and data made available on the ECHA website may be reproduced, distributed and/or used, totally or in part, for non-commercial purposes provided that ECHA is acknowledged as the source: "Source: European Chemicals Agency, http://echa.europa.eu/". Such acknowledgement must be included in each copy of the material. ECHA permits and encourages organisations and individuals to create links to the ECHA website under the following cumulative conditions: Links can only be made to webpages that provide a link to the Legal Notice page. | |
Record name | MEVALONOLACTONE, (±)- | |
Source | FDA Global Substance Registration System (GSRS) | |
URL | https://gsrs.ncats.nih.gov/ginas/app/beta/substances/1RJ06DC41B | |
Description | The FDA Global Substance Registration System (GSRS) enables the efficient and accurate exchange of information on what substances are in regulated products. Instead of relying on names, which vary across regulatory domains, countries, and regions, the GSRS knowledge base makes it possible for substances to be defined by standardized, scientific descriptions. | |
Explanation | Unless otherwise noted, the contents of the FDA website (www.fda.gov), both text and graphics, are not copyrighted. They are in the public domain and may be republished, reprinted and otherwise used freely by anyone without the need to obtain permission from FDA. Credit to the U.S. Food and Drug Administration as the source is appreciated but not required. | |
Synthesis routes and methods
Procedure details
Retrosynthesis Analysis
AI-Powered Synthesis Planning: Our tool employs the Template_relevance Pistachio, Template_relevance Bkms_metabolic, Template_relevance Pistachio_ringbreaker, Template_relevance Reaxys, Template_relevance Reaxys_biocatalysis model, leveraging a vast database of chemical reactions to predict feasible synthetic routes.
One-Step Synthesis Focus: Specifically designed for one-step synthesis, it provides concise and direct routes for your target compounds, streamlining the synthesis process.
Accurate Predictions: Utilizing the extensive PISTACHIO, BKMS_METABOLIC, PISTACHIO_RINGBREAKER, REAXYS, REAXYS_BIOCATALYSIS database, our tool offers high-accuracy predictions, reflecting the latest in chemical research and data.
Strategy Settings
Precursor scoring | Relevance Heuristic |
---|---|
Min. plausibility | 0.01 |
Model | Template_relevance |
Template Set | Pistachio/Bkms_metabolic/Pistachio_ringbreaker/Reaxys/Reaxys_biocatalysis |
Top-N result to add to graph | 6 |
Feasible Synthetic Routes
Avertissement et informations sur les produits de recherche in vitro
Veuillez noter que tous les articles et informations sur les produits présentés sur BenchChem sont destinés uniquement à des fins informatives. Les produits disponibles à l'achat sur BenchChem sont spécifiquement conçus pour des études in vitro, qui sont réalisées en dehors des organismes vivants. Les études in vitro, dérivées du terme latin "in verre", impliquent des expériences réalisées dans des environnements de laboratoire contrôlés à l'aide de cellules ou de tissus. Il est important de noter que ces produits ne sont pas classés comme médicaments et n'ont pas reçu l'approbation de la FDA pour la prévention, le traitement ou la guérison de toute condition médicale, affection ou maladie. Nous devons souligner que toute forme d'introduction corporelle de ces produits chez les humains ou les animaux est strictement interdite par la loi. Il est essentiel de respecter ces directives pour assurer la conformité aux normes légales et éthiques en matière de recherche et d'expérimentation.