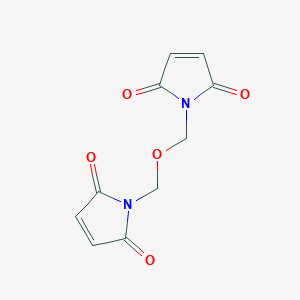
Bis-maleimidomethyl ether
Vue d'ensemble
Description
Bis-maleimidomethyl ether (CAS 15209-14-0) is a homobifunctional crosslinker with the molecular formula C₁₀H₈N₂O₅ and a molecular weight of 236.18 g/mol . It features two maleimide groups that react selectively with thiol (-SH) groups via Michael addition, forming stable covalent bonds . This compound is a white to pale-yellow solid with a melting point of 129–130°C, a density of 1.552 g/cm³, and low vapor pressure (1.11 × 10⁻⁷ mmHg at 25°C) . It is soluble in acetone, chloroform, and methanol, making it suitable for applications in polymer chemistry and bioconjugation .
Key applications include:
- Protein crosslinking: Used to dimerize enzymes like human carbonic anhydrase II (HCAII) for structural studies .
- Antisickling agent: Modifies hemoglobin by targeting β93 cysteine and β97 histidine residues, stabilizing oxygenated hemoglobin and preventing erythrocyte sickling .
- Polymer networks: Facilitates dynamic crosslinking in materials science due to rapid thiol-maleimide reactivity .
Méthodes De Préparation
Direct Acid Chloride Coupling Method
This method, detailed in WO 2000/010974A2 , involves the direct reaction of acid chloride-functionalized maleimides with hydroxyl groups on ether backbones. It is particularly suited for synthesizing mono- and multifunctional maleimide ethers.
Reaction Mechanism
N-[(Chloroformyl)methyl]maleimide reacts with diols or polyols in the presence of a base (e.g., pyridine). The hydroxyl group performs a nucleophilic attack on the acid chloride, forming an ether linkage and releasing HCl. A key advantage is the avoidance of intermediate isolation, which reduces side reactions .
Critical Process Parameters
-
Solvent Selection : Dimethyl carbonate enhances yields (up to 23% improvement compared to THF) by stabilizing the transition state .
-
Stabilizers : Radical inhibitors like tert-butyl catechol (0.1–1.0 wt%) prevent maleimide polymerization at elevated temperatures .
-
Stoichiometry : A 1:1 molar ratio of maleimide acid chloride to hydroxyl groups ensures complete functionalization without crosslinking .
Industrial Scalability Challenges
Large-scale production faces hurdles in HCl management and solvent recovery. Continuous flow reactors with in-line neutralization (e.g., NaOH scrubbers) are proposed to address these issues .
Comparative Analysis of Preparation Methods
The table below contrasts the two primary methods:
Analyse Des Réactions Chimiques
Types de réactions
Le Bis(N-maléimidométhyl)éther subit diverses réactions chimiques, notamment :
Réactions d'addition : Les groupes maléimides peuvent participer à des réactions d'addition de Michael avec des nucléophiles tels que les thiols et les amines.
Réactions de cycloaddition : Le composé peut subir des réactions de Diels-Alder avec des diènes pour former des cycloadduits.
Réactions de substitution : La liaison éther peut être clivée dans des conditions acides ou basiques pour former les dérivés maléimides correspondants.
Réactifs et conditions courantes
Nucléophiles : Les thiols, les amines et les alcools sont couramment utilisés dans les réactions d'addition.
Catalyseurs : La triéthylamine et d'autres bases sont utilisées pour catalyser les réactions.
Solvants : Le diméthylformamide, le dichlorométhane et l'acétonitrile sont fréquemment utilisés comme solvants.
Principaux produits formés
Adduits de Michael : Formés par l'addition de nucléophiles aux groupes maléimides.
Cycloadduits : Résultant de réactions de cycloaddition avec des diènes.
Dérivés maléimides : Produits à partir du clivage de la liaison éther.
Applications de la recherche scientifique
Le Bis(N-maléimidométhyl)éther a une large gamme d'applications dans la recherche scientifique, notamment :
Chimie : Utilisé comme agent de réticulation en chimie des polymères pour créer des réseaux stables et améliorer les propriétés des matériaux.
Biologie : Employé dans les études de modification et de marquage des protéines en raison de sa capacité à former des liaisons covalentes avec les résidus cystéine des protéines.
Médecine : Etudié comme agent anti-falciformation pour le traitement de la drépanocytose en stabilisant l'hémoglobine et en empêchant la falciformation des globules rouges.
Industrie : Utilisé dans la production de matériaux avancés, d'adhésifs et de revêtements grâce à ses groupes maléimides réactifs.
Mécanisme d'action
Le mécanisme d'action du Bis(N-maléimidométhyl)éther implique la formation de liaisons covalentes avec des groupes nucléophiles dans les molécules cibles. Les groupes maléimides réagissent avec les groupes thiol ou amine des protéines ou d'autres biomolécules, conduisant à la formation de liaisons covalentes stables. Cette réactivité est exploitée dans diverses applications, telles que la réticulation et la modification des protéines .
Applications De Recherche Scientifique
Biochemical Applications
1.1 Antisickling Agent
BMME has been studied for its potential as an antisickling reagent in treating sickle cell disease. It interacts with oxyhemoglobin, forming covalent linkages that stabilize hemoglobin and prevent the sickling reaction upon deoxygenation. The interaction specifically modifies cysteine and histidine residues on the beta chain of hemoglobin S, leading to a left-shifted oxyhemoglobin equilibrium curve, which abolishes cooperativity in oxygen binding .
Case Study: Hemoglobin Modification
- Objective: To determine the efficacy of BMME in preventing sickling.
- Method: BMME was introduced to red blood cells containing hemoglobin S.
- Results: The modified hemoglobin remained stable without adversely affecting red cell enzyme activities or membrane integrity.
- Conclusion: BMME effectively alters hemoglobin structure to mitigate sickling, although further research is needed to elucidate the precise mechanisms involved.
Polymer Science Applications
2.1 Synthesis of Bismaleimide Polymers
BMME serves as a precursor for synthesizing bismaleimide (BMI) polymers, which are known for their thermal stability and mechanical properties. These polymers are synthesized through the reaction of BMME with various diamines, resulting in materials suitable for high-performance applications.
Table 1: Properties of BMI Polymers Synthesized from BMME
Polymer Type | Flexural Modulus (psi) | Thermal Stability (°C) | Solubility |
---|---|---|---|
BMI from EDR-148 | ~500,000 | Up to 250 | Water-soluble |
BMI from Tetraethylene Glycol Diamine | ~460,000 | Up to 230 | Poorly soluble |
2.2 Microelectronics Applications
Recent studies have focused on the use of BMME-based BMI polymers in microelectronics due to their excellent dielectric properties and thermal stability. The incorporation of aliphatic-ether chains enhances flexibility and processability, making them suitable for advanced electronic applications .
Case Study: Microelectronics Development
- Objective: To develop BMME-based polymers for microelectronic applications.
- Method: Synthesis and characterization of BMI containing aliphatic-ether chains.
- Results: The resulting polymers exhibited improved thermal properties and dielectric constants suitable for electronic substrates.
- Conclusion: BMME-derived polymers show promise for future microelectronic devices due to their enhanced properties.
Summary of Findings
The applications of bis-maleimidomethyl ether span across biochemical and polymer science domains. Its role as an antisickling agent demonstrates significant therapeutic potential, while its utility in synthesizing high-performance polymers positions it as a valuable compound in material science.
Mécanisme D'action
The mechanism of action of Bis(N-maleimidomethyl)ether involves the formation of covalent bonds with nucleophilic groups in target molecules. The maleimide groups react with thiol or amine groups in proteins or other biomolecules, leading to the formation of stable covalent linkages. This reactivity is exploited in various applications, such as protein cross-linking and modification .
Comparaison Avec Des Composés Similaires
Structural and Functional Comparison
Table 1: Structural and Functional Properties
Reactivity and Selectivity
- This compound exhibits thiol-specific reactivity , enabling precise crosslinking in biological systems (e.g., hemoglobin modification) . In contrast, chlorinated or brominated ethers (e.g., bis-(chloromethyl) ether) act as broad alkylating agents , reacting with nucleophiles like DNA bases, which contributes to their toxicity .
- The Michael addition kinetics of maleimides (second-order rate constant ~10–100 M⁻¹s⁻¹) are faster and more selective than the SN2 mechanisms of halogenated ethers, which lack specificity .
Table 2: Hazard Profiles
This compound poses lower acute toxicity compared to halogenated analogs but requires handling precautions due to irritant properties. Chlorinated/brominated ethers are associated with carcinogenicity and systemic toxicity .
Activité Biologique
Bis-maleimidomethyl ether (BMME), a bifunctional maleimide compound, has garnered significant attention in biochemical research due to its unique ability to form covalent bonds with thiol groups in proteins. This property makes it a valuable reagent for various applications, particularly in the modification of hemoglobin and its potential therapeutic use in sickle cell disease. This article explores the biological activity of BMME, including its mechanisms, applications, and relevant case studies.
Chemical Structure and Properties
BMME is characterized by two maleimide functional groups linked by a methylene ether bridge. The compound's structure allows it to react specifically with thiol-containing biomolecules, facilitating the formation of stable covalent linkages. This reactivity is crucial for its applications in bioconjugation and protein modification.
The primary mechanism of action for BMME involves its interaction with sulfhydryl groups in proteins, particularly hemoglobin. When BMME reacts with oxyhemoglobin, it forms covalent bonds that convert maleimide into succinyl derivatives at specific cysteine and histidine residues. This modification alters the hemoglobin's properties, including its oxygen-binding affinity and cooperativity. Notably, the resultant hemoglobin exhibits a left-shifted oxyhemoglobin equilibrium curve, which is associated with increased oxygen affinity and reduced sickling propensity under deoxygenated conditions .
Biological Applications
1. Antisickling Agent
BMME has been extensively studied as an antisickling reagent due to its ability to modify hemoglobin S (Hb S) and prevent sickling under hypoxic conditions. Research indicates that BMME can traverse red cell membranes and inhibit the polymerization of deoxygenated Hb S, thus mitigating the symptoms of sickle cell disease without adversely affecting red cell enzyme activities or membrane integrity .
2. Monoclonal Antibody Production
In addition to its role in hemoglobin modification, BMME has been shown to stimulate monoclonal antibody (mAb) production in cell cultures. It enhances cell-specific glucose uptake rates and increases intracellular adenosine triphosphate (ATP) levels during mAb production processes.
3. Protein Labeling and Modification
BMME is utilized in various biochemical applications for protein labeling and modification. Its ability to form stable conjugates with thiol groups makes it a useful tool for studying protein dynamics and interactions within living systems .
Case Studies
Several studies have highlighted the efficacy of BMME in different biological contexts:
- Sickle Cell Disease Treatment : A study demonstrated that treatment with BMME significantly reduced the sickling of erythrocytes from individuals with sickle cell disease when exposed to hypoxic conditions. The modified hemoglobin displayed enhanced oxygen affinity and stability compared to untreated controls .
- Monoclonal Antibody Production : In experimental setups, BMME was found to increase the yield of mAbs by promoting metabolic activity in hybridoma cells, showcasing its potential utility in biopharmaceutical production.
Comparative Analysis
The following table summarizes key characteristics of BMME compared to similar compounds:
Compound | Structure Type | Unique Features |
---|---|---|
This compound (BMME) | Bifunctional | Effective antisickling agent; modifies hemoglobin |
Maleimide | Monofunctional | Single reactive site for thiol modification |
N-Succinimidyl Maleimide | Monofunctional | Used for protein labeling but less stable than BMME |
Bis(maleimido)ethane | Bifunctional | Similar reactivity but less effective biologically |
Safety Considerations
While BMME is a powerful reagent, it is important to handle it with care due to potential irritant effects on skin, eyes, and respiratory systems. Safety data sheets should be consulted prior to laboratory use.
Q & A
Q. Basic: What are the established methods for synthesizing and characterizing Bis-maleimidomethyl ether?
Answer: this compound is typically synthesized via nucleophilic substitution reactions. A common approach involves reacting maleic anhydride derivatives with methyl ether precursors under controlled anhydrous conditions. Characterization requires a combination of spectroscopic and crystallographic techniques:
- Nuclear Magnetic Resonance (NMR): Analyze H and C NMR spectra to confirm the maleimide and ether linkages. Peaks at δ 6.7–7.0 ppm (vinyl protons) and δ 40–50 ppm (ether carbons) are indicative .
- Mass Spectrometry (MS): Use high-resolution MS to verify molecular ion peaks (e.g., [M+H] at m/z 254.1) and fragmentation patterns .
- X-ray Crystallography: Resolve crystal structures to confirm stereochemistry and bond angles, particularly for coordination compounds derived from the ether .
Q. Basic: What analytical techniques are critical for confirming the structural integrity of this compound?
Answer: Structural validation relies on:
- Fourier-Transform Infrared Spectroscopy (FTIR): Identify maleimide C=O stretches (~1700 cm) and ether C-O-C stretches (~1100 cm) .
- Elemental Analysis: Verify purity (>98%) by matching experimental and theoretical C, H, N, and O percentages .
- Chromatography: Use HPLC with UV detection (λ = 280 nm) to assess purity and detect byproducts .
Q. Advanced: How does this compound interact with biological macromolecules like hemoglobin?
Answer: The compound covalently binds to sulfhydryl (Cys) and imidazolyl (His) residues on hemoglobin’s β-chain. Methodological approaches include:
- Spectrophotometric Assays: Monitor absorbance shifts at 540 nm (oxyhemoglobin to deoxyhemoglobin) to study equilibrium curve perturbations .
- Mass Spectrometry: Identify succinyl adducts (e.g., β93 Cys-maleimide) via peptide mapping and tandem MS .
- Circular Dichroism (CD): Assess conformational changes in hemoglobin’s secondary structure post-modification .
Q. Advanced: How can researchers resolve contradictions in reactivity data for this compound across studies?
Answer: Address discrepancies by:
- Replicating Experimental Conditions: Standardize pH, temperature, and solvent systems (e.g., DMF vs. THF) to isolate variables .
- Secondary Source Validation: Cross-reference data with peer-reviewed repositories (e.g., NIST Chemistry WebBook) to verify spectral or kinetic parameters .
- Error Analysis: Quantify uncertainties in titration endpoints or spectroscopic measurements using standard deviation calculations .
Q. Basic: What safety protocols are essential when handling this compound in laboratory settings?
Answer:
- Personal Protective Equipment (PPE): Use nitrile gloves, lab coats, and fume hoods to avoid dermal/ocular exposure .
- Incompatibility Management: Avoid strong acids/oxidizers (e.g., HNO) to prevent hazardous decomposition (e.g., toxic fumes) .
- Waste Disposal: Neutralize residues with 10% sodium bicarbonate before disposal in designated organic waste containers .
Q. Advanced: What experimental designs are effective for studying this compound’s catalytic applications?
Answer:
- Coordination Chemistry Studies: Synthesize metal complexes (e.g., Cu or Zn) and evaluate catalytic efficiency in reactions like the Henry reaction. Monitor turnover frequency (TOF) via gas chromatography .
- Kinetic Profiling: Use stopped-flow spectroscopy to measure reaction rates under varying ligand concentrations .
- Computational Modeling: Apply DFT calculations (e.g., Gaussian 09) to predict binding affinities and transition states .
Q. Basic: What strategies are recommended for conducting a systematic literature review on this compound?
Answer:
- Database Selection: Prioritize PubMed, TOXLINE, and SciFinder for toxicological and synthetic data .
- Search Strings: Use Boolean operators (e.g., "this compound" AND "antisickling") and MeSH terms (e.g., "Hemoglobin S/chemistry") .
- Two-Step Screening: Filter titles/abstracts first, then perform full-text reviews to exclude irrelevant studies .
Q. Advanced: How should statistical methods be applied in toxicological studies of this compound?
Answer:
- Dose-Response Modeling: Use probit or logit regression to calculate LD values from acute toxicity assays .
- Confounding Factor Control: Apply ANOVA to isolate effects of variables like solvent choice or exposure duration .
- Meta-Analysis: Aggregate data from multiple studies using random-effects models to account for heterogeneity .
Advanced: What mechanistic questions remain unresolved about this compound’s antisickling effects?
Answer: Key unknowns include:
- Cooperativity Loss: Use single-molecule FRET to study how covalent modifications disrupt hemoglobin’s allosteric transitions .
- Membrane Permeability: Employ fluorescence microscopy with liposome models to quantify transport kinetics .
- Enzyme Interactions: Perform activity assays (e.g., G6PDH) to rule out off-target effects on red cell enzymes .
Q. Advanced: How can researchers address inconsistencies in toxicological data for this compound?
Answer:
- Source Evaluation: Prioritize studies with explicit methodologies (e.g., ATSDR toxicological profiles) over anecdotal reports .
- In Silico Predictions: Use tools like EPA’s TEST to estimate toxicity endpoints when experimental data are lacking .
- Interlaboratory Comparisons: Participate in round-robin studies to calibrate assay protocols and reduce variability .
Propriétés
IUPAC Name |
1-[(2,5-dioxopyrrol-1-yl)methoxymethyl]pyrrole-2,5-dione | |
---|---|---|
Source | PubChem | |
URL | https://pubchem.ncbi.nlm.nih.gov | |
Description | Data deposited in or computed by PubChem | |
InChI |
InChI=1S/C10H8N2O5/c13-7-1-2-8(14)11(7)5-17-6-12-9(15)3-4-10(12)16/h1-4H,5-6H2 | |
Source | PubChem | |
URL | https://pubchem.ncbi.nlm.nih.gov | |
Description | Data deposited in or computed by PubChem | |
InChI Key |
UTRLJOWPWILGSB-UHFFFAOYSA-N | |
Source | PubChem | |
URL | https://pubchem.ncbi.nlm.nih.gov | |
Description | Data deposited in or computed by PubChem | |
Canonical SMILES |
C1=CC(=O)N(C1=O)COCN2C(=O)C=CC2=O | |
Source | PubChem | |
URL | https://pubchem.ncbi.nlm.nih.gov | |
Description | Data deposited in or computed by PubChem | |
Molecular Formula |
C10H8N2O5 | |
Source | PubChem | |
URL | https://pubchem.ncbi.nlm.nih.gov | |
Description | Data deposited in or computed by PubChem | |
DSSTOX Substance ID |
DTXSID30934420 | |
Record name | 1,1'-[Oxybis(methylene)]di(1H-pyrrole-2,5-dione) | |
Source | EPA DSSTox | |
URL | https://comptox.epa.gov/dashboard/DTXSID30934420 | |
Description | DSSTox provides a high quality public chemistry resource for supporting improved predictive toxicology. | |
Molecular Weight |
236.18 g/mol | |
Source | PubChem | |
URL | https://pubchem.ncbi.nlm.nih.gov | |
Description | Data deposited in or computed by PubChem | |
CAS No. |
15209-14-0 | |
Record name | Bis(N-maleimidomethyl)ether | |
Source | ChemIDplus | |
URL | https://pubchem.ncbi.nlm.nih.gov/substance/?source=chemidplus&sourceid=0015209140 | |
Description | ChemIDplus is a free, web search system that provides access to the structure and nomenclature authority files used for the identification of chemical substances cited in National Library of Medicine (NLM) databases, including the TOXNET system. | |
Record name | Bis(N-maleimidomethyl)ether | |
Source | DrugBank | |
URL | https://www.drugbank.ca/drugs/DB04085 | |
Description | The DrugBank database is a unique bioinformatics and cheminformatics resource that combines detailed drug (i.e. chemical, pharmacological and pharmaceutical) data with comprehensive drug target (i.e. sequence, structure, and pathway) information. | |
Explanation | Creative Common's Attribution-NonCommercial 4.0 International License (http://creativecommons.org/licenses/by-nc/4.0/legalcode) | |
Record name | 1,1'-[Oxybis(methylene)]di(1H-pyrrole-2,5-dione) | |
Source | EPA DSSTox | |
URL | https://comptox.epa.gov/dashboard/DTXSID30934420 | |
Description | DSSTox provides a high quality public chemistry resource for supporting improved predictive toxicology. | |
Record name | Bis-maleimidomethylether | |
Source | European Chemicals Agency (ECHA) | |
URL | https://echa.europa.eu/information-on-chemicals | |
Description | The European Chemicals Agency (ECHA) is an agency of the European Union which is the driving force among regulatory authorities in implementing the EU's groundbreaking chemicals legislation for the benefit of human health and the environment as well as for innovation and competitiveness. | |
Explanation | Use of the information, documents and data from the ECHA website is subject to the terms and conditions of this Legal Notice, and subject to other binding limitations provided for under applicable law, the information, documents and data made available on the ECHA website may be reproduced, distributed and/or used, totally or in part, for non-commercial purposes provided that ECHA is acknowledged as the source: "Source: European Chemicals Agency, http://echa.europa.eu/". Such acknowledgement must be included in each copy of the material. ECHA permits and encourages organisations and individuals to create links to the ECHA website under the following cumulative conditions: Links can only be made to webpages that provide a link to the Legal Notice page. | |
Record name | BIS(N-MALEIMIDOMETHYL) ETHER | |
Source | FDA Global Substance Registration System (GSRS) | |
URL | https://gsrs.ncats.nih.gov/ginas/app/beta/substances/5AO12W9Y4D | |
Description | The FDA Global Substance Registration System (GSRS) enables the efficient and accurate exchange of information on what substances are in regulated products. Instead of relying on names, which vary across regulatory domains, countries, and regions, the GSRS knowledge base makes it possible for substances to be defined by standardized, scientific descriptions. | |
Explanation | Unless otherwise noted, the contents of the FDA website (www.fda.gov), both text and graphics, are not copyrighted. They are in the public domain and may be republished, reprinted and otherwise used freely by anyone without the need to obtain permission from FDA. Credit to the U.S. Food and Drug Administration as the source is appreciated but not required. | |
Retrosynthesis Analysis
AI-Powered Synthesis Planning: Our tool employs the Template_relevance Pistachio, Template_relevance Bkms_metabolic, Template_relevance Pistachio_ringbreaker, Template_relevance Reaxys, Template_relevance Reaxys_biocatalysis model, leveraging a vast database of chemical reactions to predict feasible synthetic routes.
One-Step Synthesis Focus: Specifically designed for one-step synthesis, it provides concise and direct routes for your target compounds, streamlining the synthesis process.
Accurate Predictions: Utilizing the extensive PISTACHIO, BKMS_METABOLIC, PISTACHIO_RINGBREAKER, REAXYS, REAXYS_BIOCATALYSIS database, our tool offers high-accuracy predictions, reflecting the latest in chemical research and data.
Strategy Settings
Precursor scoring | Relevance Heuristic |
---|---|
Min. plausibility | 0.01 |
Model | Template_relevance |
Template Set | Pistachio/Bkms_metabolic/Pistachio_ringbreaker/Reaxys/Reaxys_biocatalysis |
Top-N result to add to graph | 6 |
Feasible Synthetic Routes
Avertissement et informations sur les produits de recherche in vitro
Veuillez noter que tous les articles et informations sur les produits présentés sur BenchChem sont destinés uniquement à des fins informatives. Les produits disponibles à l'achat sur BenchChem sont spécifiquement conçus pour des études in vitro, qui sont réalisées en dehors des organismes vivants. Les études in vitro, dérivées du terme latin "in verre", impliquent des expériences réalisées dans des environnements de laboratoire contrôlés à l'aide de cellules ou de tissus. Il est important de noter que ces produits ne sont pas classés comme médicaments et n'ont pas reçu l'approbation de la FDA pour la prévention, le traitement ou la guérison de toute condition médicale, affection ou maladie. Nous devons souligner que toute forme d'introduction corporelle de ces produits chez les humains ou les animaux est strictement interdite par la loi. Il est essentiel de respecter ces directives pour assurer la conformité aux normes légales et éthiques en matière de recherche et d'expérimentation.