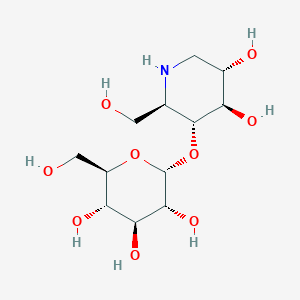
4-O-alpha-D-Glucopyranosylmoranoline
Vue d'ensemble
Description
4-O-alpha-D-Glucopyranosylmoranoline is a monosaccharide derivative characterized by the presence of an alpha-D-glucosyl residue attached at position 4 of a (2R,3R,4R,5S)-2-(hydroxymethyl)piperidine-3,4,5-triol structure . This compound is known for its role as a metabolite and its involvement in various biological processes .
Applications De Recherche Scientifique
4-O-alpha-D-Glucopyranosylmoranoline has a wide range of applications in scientific research:
Méthodes De Préparation
Synthetic Routes and Reaction Conditions
The synthesis of 4-O-alpha-D-Glucopyranosylmoranoline typically involves a transglycosylation reaction. This reaction uses N-substituted moranolines and soluble starch as the glucose donor, catalyzed by cyclodextrin glycosyltransferase from Bacillus stearothermophilus . The resultant transglycosylation products are then hydrolyzed by glucoamylase from Rhizopus niveus . This enzymatic synthesis method is notable for its efficiency, converting a significant percentage of the starting materials into the desired product .
Industrial Production Methods
Industrial production of this compound follows similar enzymatic synthesis routes, leveraging large-scale bioreactors to facilitate the transglycosylation and hydrolysis reactions. The use of biocatalysts ensures high specificity and yield, making the process economically viable for large-scale production .
Analyse Des Réactions Chimiques
Types of Reactions
4-O-alpha-D-Glucopyranosylmoranoline primarily undergoes glycosidic bond formation and hydrolysis reactions. It can also participate in oxidation and reduction reactions due to the presence of hydroxyl groups in its structure .
Common Reagents and Conditions
Transglycosylation: Catalyzed by cyclodextrin glycosyltransferase, using soluble starch as the glucose donor.
Hydrolysis: Catalyzed by glucoamylase, breaking down the transglycosylation products into the final compound.
Major Products
The major product of these reactions is this compound itself, with potential minor by-products depending on the specific reaction conditions and substrates used .
Mécanisme D'action
4-O-alpha-D-Glucopyranosylmoranoline exerts its effects primarily through the inhibition of alpha-glucosidase, an enzyme involved in the breakdown of carbohydrates into glucose . By inhibiting this enzyme, the compound can reduce the rate of glucose absorption in the intestines, thereby helping to regulate blood sugar levels . The molecular targets include the active sites of alpha-glucosidase, where the compound binds and prevents the enzyme from catalyzing its substrate .
Comparaison Avec Des Composés Similaires
4-O-alpha-D-Glucopyranosylmoranoline is similar to other alpha-glucosidase inhibitors such as 1-deoxynojirimycin (DNJ) and its derivatives . it is unique in its specific glycosidic linkage and the presence of an alpha-D-glucosyl residue, which confer distinct inhibitory properties and metabolic stability . Other similar compounds include N-hydroxyethyl DNJ and N-butyl DNJ, which also inhibit alpha-glucosidase but differ in their side chain modifications and potency .
Activité Biologique
4-O-alpha-D-Glucopyranosylmoranoline is a notable monosaccharide derivative that has garnered attention for its biological activities, particularly in the context of glycosidase inhibition and potential therapeutic applications. This article explores the compound's biological activity, focusing on its mechanisms of action, synthesis methods, and relevant case studies that highlight its efficacy in various applications.
Chemical Structure and Properties
This compound is characterized by an alpha-D-glucosyl residue attached to a (2R,3R,4R,5S)-2-(hydroxymethyl)piperidine-3,4,5-triol structure. Its molecular weight is approximately 325.31 g/mol . The compound primarily exists as a solid and is recognized for its stability under various conditions.
Property | Value |
---|---|
Molecular Formula | C₁₄H₂₃O₇ |
Molecular Weight | 325.31 g/mol |
Physical State | Solid |
Solubility | Soluble in water |
This compound primarily exerts its biological effects through the inhibition of alpha-glucosidase, an enzyme crucial for carbohydrate metabolism. By inhibiting this enzyme, the compound slows down the breakdown of complex carbohydrates into glucose, thereby regulating blood glucose levels effectively. This mechanism positions it as a potential hypoglycemic agent .
Therapeutic Applications
The compound has been investigated for various therapeutic applications:
- Hypoglycemic Effects : Studies have shown that this compound can significantly lower blood glucose levels in animal models, making it a candidate for diabetes management .
- Enzyme Inhibition : It serves as a model compound in research focused on glycosidase inhibition and carbohydrate metabolism, which could have implications for developing treatments for metabolic disorders .
Case Studies
Several case studies have explored the efficacy of this compound in clinical settings:
- Diabetes Management : A study involving diabetic rats demonstrated that administration of this compound led to a significant reduction in postprandial blood glucose levels compared to control groups. The results indicated an enhancement in insulin sensitivity and overall glycemic control .
- Metabolic Pathways : Another investigation focused on the metabolic pathways influenced by this compound revealed alterations in glucose metabolism markers, suggesting potential benefits in managing metabolic syndrome .
- Glycosidase Inhibition : Research highlighted its effectiveness as an alpha-glucosidase inhibitor compared to traditional drugs like acarbose, showcasing its potential as a safer alternative with fewer side effects .
Table 2: Summary of Case Studies on this compound
Study Focus | Findings |
---|---|
Diabetes Management | Significant reduction in postprandial glucose levels |
Metabolic Pathways | Altered glucose metabolism markers |
Glycosidase Inhibition | More effective than traditional alpha-glucosidase inhibitors |
Synthesis and Production
The synthesis of this compound typically involves enzymatic transglycosylation reactions. This process uses N-substituted moranolines and soluble starch as glucose donors, catalyzed by cyclodextrin glycosyltransferase from Bacillus stearothermophilus. The industrial production leverages biocatalysts to ensure high specificity and yield, making it economically viable for large-scale applications.
Table 3: Synthesis Methods
Method | Description |
---|---|
Enzymatic Transglycosylation | Utilizes cyclodextrin glycosyltransferase for synthesis |
Industrial Production | Large-scale bioreactor processes with biocatalysts |
Propriétés
IUPAC Name |
(2S,3R,4S,5S,6R)-2-[(2R,3R,4R,5S)-4,5-dihydroxy-2-(hydroxymethyl)piperidin-3-yl]oxy-6-(hydroxymethyl)oxane-3,4,5-triol | |
---|---|---|
Source | PubChem | |
URL | https://pubchem.ncbi.nlm.nih.gov | |
Description | Data deposited in or computed by PubChem | |
InChI |
InChI=1S/C12H23NO9/c14-2-4-11(7(17)5(16)1-13-4)22-12-10(20)9(19)8(18)6(3-15)21-12/h4-20H,1-3H2/t4-,5+,6-,7-,8-,9+,10-,11-,12-/m1/s1 | |
Source | PubChem | |
URL | https://pubchem.ncbi.nlm.nih.gov | |
Description | Data deposited in or computed by PubChem | |
InChI Key |
GNVIYGFSOIHFHK-NIKVEEOSSA-N | |
Source | PubChem | |
URL | https://pubchem.ncbi.nlm.nih.gov | |
Description | Data deposited in or computed by PubChem | |
Canonical SMILES |
C1C(C(C(C(N1)CO)OC2C(C(C(C(O2)CO)O)O)O)O)O | |
Source | PubChem | |
URL | https://pubchem.ncbi.nlm.nih.gov | |
Description | Data deposited in or computed by PubChem | |
Isomeric SMILES |
C1[C@@H]([C@H]([C@@H]([C@H](N1)CO)O[C@@H]2[C@@H]([C@H]([C@@H]([C@H](O2)CO)O)O)O)O)O | |
Source | PubChem | |
URL | https://pubchem.ncbi.nlm.nih.gov | |
Description | Data deposited in or computed by PubChem | |
Molecular Formula |
C12H23NO9 | |
Source | PubChem | |
URL | https://pubchem.ncbi.nlm.nih.gov | |
Description | Data deposited in or computed by PubChem | |
DSSTOX Substance ID |
DTXSID701135748 | |
Record name | (2R,3R,4R,5S)-4,5-Dihydroxy-2-(hydroxymethyl)-3-piperidinyl α-D-glucopyranoside | |
Source | EPA DSSTox | |
URL | https://comptox.epa.gov/dashboard/DTXSID701135748 | |
Description | DSSTox provides a high quality public chemistry resource for supporting improved predictive toxicology. | |
Molecular Weight |
325.31 g/mol | |
Source | PubChem | |
URL | https://pubchem.ncbi.nlm.nih.gov | |
Description | Data deposited in or computed by PubChem | |
Physical Description |
Solid | |
Record name | Glucopyranosylmoranoline | |
Source | Human Metabolome Database (HMDB) | |
URL | http://www.hmdb.ca/metabolites/HMDB0059718 | |
Description | The Human Metabolome Database (HMDB) is a freely available electronic database containing detailed information about small molecule metabolites found in the human body. | |
Explanation | HMDB is offered to the public as a freely available resource. Use and re-distribution of the data, in whole or in part, for commercial purposes requires explicit permission of the authors and explicit acknowledgment of the source material (HMDB) and the original publication (see the HMDB citing page). We ask that users who download significant portions of the database cite the HMDB paper in any resulting publications. | |
CAS No. |
80312-32-9 | |
Record name | (2R,3R,4R,5S)-4,5-Dihydroxy-2-(hydroxymethyl)-3-piperidinyl α-D-glucopyranoside | |
Source | CAS Common Chemistry | |
URL | https://commonchemistry.cas.org/detail?cas_rn=80312-32-9 | |
Description | CAS Common Chemistry is an open community resource for accessing chemical information. Nearly 500,000 chemical substances from CAS REGISTRY cover areas of community interest, including common and frequently regulated chemicals, and those relevant to high school and undergraduate chemistry classes. This chemical information, curated by our expert scientists, is provided in alignment with our mission as a division of the American Chemical Society. | |
Explanation | The data from CAS Common Chemistry is provided under a CC-BY-NC 4.0 license, unless otherwise stated. | |
Record name | (2R,3R,4R,5S)-4,5-Dihydroxy-2-(hydroxymethyl)-3-piperidinyl α-D-glucopyranoside | |
Source | EPA DSSTox | |
URL | https://comptox.epa.gov/dashboard/DTXSID701135748 | |
Description | DSSTox provides a high quality public chemistry resource for supporting improved predictive toxicology. | |
Record name | Glucopyranosylmoranoline | |
Source | Human Metabolome Database (HMDB) | |
URL | http://www.hmdb.ca/metabolites/HMDB0059718 | |
Description | The Human Metabolome Database (HMDB) is a freely available electronic database containing detailed information about small molecule metabolites found in the human body. | |
Explanation | HMDB is offered to the public as a freely available resource. Use and re-distribution of the data, in whole or in part, for commercial purposes requires explicit permission of the authors and explicit acknowledgment of the source material (HMDB) and the original publication (see the HMDB citing page). We ask that users who download significant portions of the database cite the HMDB paper in any resulting publications. | |
Retrosynthesis Analysis
AI-Powered Synthesis Planning: Our tool employs the Template_relevance Pistachio, Template_relevance Bkms_metabolic, Template_relevance Pistachio_ringbreaker, Template_relevance Reaxys, Template_relevance Reaxys_biocatalysis model, leveraging a vast database of chemical reactions to predict feasible synthetic routes.
One-Step Synthesis Focus: Specifically designed for one-step synthesis, it provides concise and direct routes for your target compounds, streamlining the synthesis process.
Accurate Predictions: Utilizing the extensive PISTACHIO, BKMS_METABOLIC, PISTACHIO_RINGBREAKER, REAXYS, REAXYS_BIOCATALYSIS database, our tool offers high-accuracy predictions, reflecting the latest in chemical research and data.
Strategy Settings
Precursor scoring | Relevance Heuristic |
---|---|
Min. plausibility | 0.01 |
Model | Template_relevance |
Template Set | Pistachio/Bkms_metabolic/Pistachio_ringbreaker/Reaxys/Reaxys_biocatalysis |
Top-N result to add to graph | 6 |
Feasible Synthetic Routes
Avertissement et informations sur les produits de recherche in vitro
Veuillez noter que tous les articles et informations sur les produits présentés sur BenchChem sont destinés uniquement à des fins informatives. Les produits disponibles à l'achat sur BenchChem sont spécifiquement conçus pour des études in vitro, qui sont réalisées en dehors des organismes vivants. Les études in vitro, dérivées du terme latin "in verre", impliquent des expériences réalisées dans des environnements de laboratoire contrôlés à l'aide de cellules ou de tissus. Il est important de noter que ces produits ne sont pas classés comme médicaments et n'ont pas reçu l'approbation de la FDA pour la prévention, le traitement ou la guérison de toute condition médicale, affection ou maladie. Nous devons souligner que toute forme d'introduction corporelle de ces produits chez les humains ou les animaux est strictement interdite par la loi. Il est essentiel de respecter ces directives pour assurer la conformité aux normes légales et éthiques en matière de recherche et d'expérimentation.