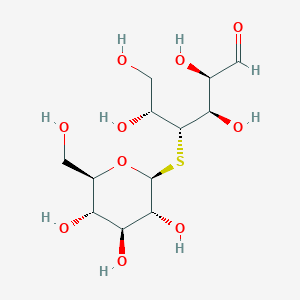
D-Glucose, 4-S-beta-D-glucopyranosyl-4-thio-
Vue d'ensemble
Description
D-Glucose, 4-S-β-D-glucopyranosyl-4-thio- (synonyms: Thiocellobiose, TCB) is a thioglycoside derivative of cellobiose, where the oxygen atom in the β-1,4-glycosidic bond is replaced by a sulfur atom. Its IUPAC name is (2R,3S,4S,5R,6S)-2-(hydroxymethyl)-6-[(2R,3S,4R,5R,6R)-4,5,6-trihydroxy-2-(hydroxymethyl)oxan-3-yl]sulfanyloxane-3,4,5-triol . Structurally, it consists of two β-D-glucopyranose units linked via a thioether bond at the C-4 position (Figure 1).
Thiocellobiose is synthesized through chemical modifications of native cellobiose, often involving substitution reactions that introduce sulfur at the glycosidic position. Its identification is confirmed via techniques such as acid hydrolysis, TLC, and HPLC, where its retention times and derivatives align with authentic D-glucose standards . Unlike oxygen-based glycosides, the sulfur substitution alters electronic properties, solubility, and biological interactions, making it a unique compound for studying carbohydrate chemistry and enzyme specificity.
Méthodes De Préparation
β-Glycosylthiosulfonate-Mediated Synthesis
The foundational strategy for synthesizing 4-S-β-D-glucopyranosyl-4-thio-D-glucose involves β-glycosylthiosulfonate intermediates reacting with carbohydrate-derived thiol or selenol nucleophiles . This method, pioneered by early carbohydrate chemists, exploits the nucleophilic displacement of the thiosulfonate group (R-SO₂-SR') to form the sulfur-sulfur or sulfur-selenium linkage.
Reaction Mechanism and Optimization
The synthesis begins with the preparation of a β-glycosylthiosulfonate donor, typically derived from per-O-acetylated or per-O-benzoylated glucose. For example, methyl 2,3,6-tri-O-benzoyl-4-S-cyano-4-thio-α-D-glucopyranoside serves as a key intermediate . Treatment with sodium methoxide generates a thiolate ion, which undergoes nucleophilic attack on a galactopyranosyl bromide donor (e.g., 2,3,4,6-tetra-O-acetyl-α-D-galactopyranosyl bromide) in hexamethylphosphoric triamide (HMPA) . This solvent facilitates SN2 displacement by reducing ion pairing and stabilizing the transition state.
Yields for this step range from 40% to 65%, depending on the protecting group strategy. Benzoyl groups are preferred over acetyl groups due to their superior electron-withdrawing effects, which enhance the leaving group ability of the thiosulfonate . Post-coupling, deprotection via Zemplén transesterification (NaOMe/MeOH) affords the free thioglycoside.
Disulfide-Bridged Intermediate Strategy
Recent advancements by Lima et al. (2023) introduced a disulfide-mediated route to access both 4-thio-D-glucopyranose and its furanose isomer . This method addresses challenges in isolating the pyranose form, which thermodynamically favors conversion to the furanose.
Synthesis of Bis(4-thio-D-glucopyranoside)-4,4'-Disulfide
The protocol starts with 4-thio-D-glucopyranose protected as its per-O-acetylated thioglycoside. Oxidative coupling using iodine in dichloromethane generates the disulfide-bridged dimer . Key parameters include:
Parameter | Optimal Value | Effect on Yield |
---|---|---|
Oxidant | I₂ (1.1 eq) | Maximizes dimerization (78%) |
Solvent | CH₂Cl₂ | Prevents over-oxidation |
Temperature | 0°C → rt | Controls exotherm |
Dithiothreitol (DTT)-Controlled Reduction
The disulfide undergoes regioselective reduction with DTT (10 eq) in ammonium bicarbonate buffer (pH 8.3) . Monitoring by ¹H NMR reveals complete conversion to 4-thio-D-glucofuranose within 24 hours at 37°C. This method achieves a 92% yield of the furanose, with trace amounts (<5%) of the pyranose form.
Two-Route Synthesis for Thiolactose Analogues
Horton and colleagues developed parallel routes to 4-S-β-D-galactopyranosyl-4-thio-D-glucopyranose (thiolactose), adaptable to the target compound .
Route A: Direct Glycosylation
-
Donor Preparation : 2,3,4,6-Tetra-O-acetyl-α-D-galactopyranosyl bromide is activated with silver triflate in anhydrous DCM.
-
Acceptor Synthesis : Methyl 4-thio-α-D-glucopyranoside, generated from methyl α-D-galactopyranoside via triflate displacement with thiocyanate, is deprotected to the thiolate.
-
Coupling : The thiolate attacks the galactosyl donor at −40°C, yielding the protected thioglycoside in 58% yield .
Route B: 1,6-Anhydro Intermediate
-
Ring-Opening : 1,6-Anhydro-β-D-galactopyranose diacetate reacts with sodium 4-thio-glucopyranoside in HMPA.
-
Displacement : The C-4 triflate is displaced by the thiolate nucleophile, forming a 1,6-anhydro-thio-disaccharide intermediate .
-
Acetolysis : Treatment with acetic anhydride/H₂SO₄ cleaves the anhydro ring, followed by Zemplén deprotection to afford the target compound in 44% overall yield .
Comparative Analysis of Synthetic Methods
Yield and Scalability
Method | Average Yield | Scalability | Key Limitation |
---|---|---|---|
Thiosulfonate route | 55% | Moderate | Requires toxic HMPA |
Disulfide strategy | 78% (dimer) | High | Furanose predominance |
Two-route synthesis | 51% | Low | Multi-step purification |
Stereochemical Control
The β-configuration is ensured by:
-
Inverse addition (donor to acceptor) to prevent α-anomer formation
-
Participating groups at C-2 (e.g., benzoyl) that stabilize the oxocarbenium ion intermediate
-
Low-temperature conditions (−40°C) to minimize anomerization
Challenges and Mitigation Strategies
Thermodynamic Instability
The pyranose form spontaneously converts to the furanose due to reduced ring strain in the 5-membered ring . Stabilization approaches include:
-
Cryogenic storage (−80°C) under inert atmosphere
-
In situ generation using disulfide precursors immediately before use
Purification Difficulties
Thioglycosides exhibit poor crystallization. Solutions involve:
Applications De Recherche Scientifique
Biochemical Research
1.1. Enzyme Inhibition Studies
One of the primary applications of D-Glucose, 4-S-beta-D-glucopyranosyl-4-thio- is in enzyme inhibition studies. The compound acts as a substrate and inhibitor for various glycoside hydrolases, particularly cellulases. Its thioether bond enhances its stability and specificity, making it a useful tool for investigating enzyme mechanisms and kinetics. For instance, studies have shown that derivatives of this compound can effectively inhibit endoglucanases from Clostridium cellulolyticum, which are crucial for cellulose degradation .
1.2. Glycosylation Reactions
This compound is also employed in glycosylation reactions to synthesize various glycosides and oligosaccharides. Its unique structure allows for the introduction of sulfur into carbohydrate structures, leading to novel compounds with potential biological activities. Researchers have utilized it to create thio-glycosides that exhibit enhanced binding properties to lectins and other carbohydrate-binding proteins .
Drug Development
2.1. Antimicrobial Agents
D-Glucose, 4-S-beta-D-glucopyranosyl-4-thio- has been investigated for its potential as an antimicrobial agent. The thioether modification can increase the lipophilicity of the molecule, enhancing its ability to penetrate bacterial membranes. Preliminary studies suggest that this compound may exhibit activity against certain Gram-positive bacteria by disrupting their cell wall synthesis .
2.2. Cancer Therapeutics
In cancer research, compounds derived from D-Glucose, 4-S-beta-D-glucopyranosyl-4-thio- have shown promise in targeting specific cancer cell types. By modifying the sugar moiety, researchers can create targeted drug delivery systems that preferentially accumulate in tumor tissues due to the overexpression of glucose transporters in cancer cells .
Glycoscience Applications
3.1. Glycobiology Studies
The compound plays a significant role in glycobiology, where it is used to study glycan structures and their interactions with proteins. Its thioether linkage allows researchers to probe the structural requirements for glycan-protein interactions more effectively than traditional glucopyranosides .
3.2. Cellulose Research
In cellulose research, D-Glucose, 4-S-beta-D-glucopyranosyl-4-thio- serves as a model substrate for studying cellulose-degrading enzymes. Its unique bond structure provides insights into how enzymes recognize and process polysaccharides, aiding in the development of more efficient biofuels from lignocellulosic biomass .
Case Studies
Mécanisme D'action
Thiocellobiose exerts its effects primarily through its interaction with enzymes. The sulfur atom in the glycosidic linkage alters the compound’s binding affinity and specificity for certain enzymes, such as glucosidases. This interaction can inhibit enzyme activity, making thiocellobiose a valuable tool for studying enzyme mechanisms and developing enzyme inhibitors.
Comparaison Avec Des Composés Similaires
Structural and Functional Analogues
Cellobiose (β-1,4-O-D-Glucopyranosyl-D-Glucose)
- Glycosidic Bond : Oxygen-based β-1,4 linkage.
- Solubility : Highly water-soluble due to strong hydrogen bonding.
- Biological Role : Primary structural unit of cellulose; hydrolyzed by cellulases.
- Electronic Properties : Oxygen’s electronegativity facilitates stronger hydrogen bonding compared to sulfur in Thiocellobiose. Experimental and computational studies show that O-glycosides like cellobiose exhibit shorter bond lengths (C–O: ~1.43 Å) versus S-glycosides (C–S: ~1.81 Å), impacting conformational stability .
3-O-Methyl-D-Glucose (3OMG)
- Modification : Methyl group at C-3 hydroxyl.
- Functional Difference : Unlike Thiocellobiose, 3OMG is actively transported in the small intestine and used in MRI glucoCEST imaging. Its CEST contrast pH dependence is opposite to D-glucose, with higher activity at neutral pH .
4-Amino-4-Deoxy-β-D-Glucose
- Modification: Amino group replaces C-4 hydroxyl.
- Biological Activity: Acts as a glycosidase inhibitor and drug candidate (DrugBank ID: DB03291). The amino group introduces basicity, altering solubility and enzyme recognition compared to Thiocellobiose’s nonpolar sulfur .
4-Azido-4-Deoxy-β-D-Glucose
- Modification : Azido group at C-3.
- Applications : Used in click chemistry for bioconjugation. The azide group’s reactivity contrasts with Thiocellobiose’s inert thioether bond .
Physicochemical Properties
Key Research Findings
- Electronic Structure : DFT studies reveal Thiocellobiose’s bandgap and density of states (DOS) differ minimally from cellobiose, but sulfur’s larger atomic radius reduces hydrogen-bonding capacity .
- Acid Hydrolysis : Thiocellobiose requires harsher conditions (e.g., concentrated HCl) for hydrolysis compared to cellobiose, confirming sulfur’s stabilizing effect .
Activité Biologique
D-Glucose, 4-S-beta-D-glucopyranosyl-4-thio-, commonly referred to as thiocellobiose, is a unique disaccharide characterized by a sulfur linkage instead of the typical oxygen linkage found in cellobiose. This structural modification endows thiocellobiose with distinct biological activities, particularly in its interactions with enzymes involved in carbohydrate metabolism. This article presents a comprehensive overview of the biological activity of thiocellobiose, including its mechanism of action, pharmacokinetics, biochemical properties, and potential applications in research and medicine.
Overview
Thiocellobiose's chemical structure is pivotal to its biological function. As a thioglycoside, it serves as a model compound for studying the behavior of similar compounds and their interactions with various biological targets. The primary target for thiocellobiose includes glycoside hydrolases, which are enzymes that catalyze the hydrolysis of glycosidic bonds in carbohydrates.
Target Enzymes : Thiocellobiose primarily interacts with glycoside hydrolases. By binding to the active sites of these enzymes, it can inhibit their activity, thereby influencing carbohydrate metabolism pathways.
Mode of Action : The binding mechanism typically involves non-covalent interactions, such as hydrogen bonding and hydrophobic interactions. These interactions can lead to conformational changes in the enzyme that reduce its catalytic efficiency.
Pharmacokinetics
Thiocellobiose is expected to be absorbed in the digestive tract and distributed throughout the body via the bloodstream. Its metabolic stability has been demonstrated through various studies, indicating that it can maintain its structure under physiological conditions while still exerting biological effects.
The biochemical properties of thiocellobiose are not fully characterized due to limited research. However, it is known that glucose derivatives like thiocellobiose can interact with various biomolecules:
- Enzyme Inhibition : Thiocellobiose has been shown to inhibit certain glucosidases, making it a valuable tool for studying enzyme mechanisms and carbohydrate metabolism .
- Cellular Effects : Glucose derivatives play crucial roles in cellular processes such as signaling pathways and gene expression .
Case Studies and Research Findings
- Inhibition Studies : Research has indicated that thiocellobiose effectively inhibits glycosylases, which are critical in carbohydrate catabolism. This inhibition has therapeutic implications for conditions such as diabetes .
- Biodistribution Studies : In animal models, biodistribution studies revealed that thiocellobiose exhibits rapid clearance from the bloodstream with significant uptake in the intestines and bile . This suggests potential applications in drug delivery systems targeting gastrointestinal disorders.
- Metabolic Pathways : Thiocellobiose participates in various metabolic pathways related to carbohydrate metabolism. It can influence enzyme activity at different dosages, indicating a dose-dependent effect on metabolic processes .
Applications
Thiocellobiose has several promising applications across different fields:
- Research : It serves as a model compound for studying thioglycosides and their interactions with enzymes .
- Medicine : Ongoing research explores its potential therapeutic applications in enzyme inhibition and drug design .
- Industry : Thiocellobiose is utilized in biosensor development and enzyme assays due to its unique properties .
Q & A
Basic Research Questions
Q. What are the standard synthetic routes for 4-S-β-D-glucopyranosyl-4-thio-D-glucose, and how can reaction conditions influence stereochemical outcomes?
- Methodology : The synthesis of 4-thio analogues of glucopyranosides typically involves nucleophilic displacement of sulfonate derivatives (e.g., tosyl or mesyl groups) at the C4 position with sulfur-containing nucleophiles. For example, 4-thio glycosides can be synthesized via Mitsunobu reactions using 2,3,4,6-tetra-O-acetyl-D-glucopyranosyl donors under controlled anhydrous conditions to favor β-anomer formation .
- Critical Parameters : Reaction temperature, solvent polarity (e.g., DMF vs. THF), and protecting group strategy (e.g., chloroacetyl vs. benzoyl) significantly impact regioselectivity and yield. Characterization requires NMR (¹H/¹³C) and mass spectrometry to confirm sulfur substitution and anomeric configuration .
Q. How can researchers validate the purity and structural integrity of 4-S-β-D-glucopyranosyl-4-thio-D-glucose post-synthesis?
- Analytical Workflow :
Chromatography : Use reverse-phase HPLC with a C18 column and UV detection (λ = 210–230 nm for thioglycosides).
Spectroscopy : Assign ¹H-NMR peaks for the anomeric proton (δ 4.8–5.2 ppm for β-configuration) and confirm sulfur substitution via ¹³C-NMR (C4-S resonance at δ 35–40 ppm) .
Mass Spectrometry : ESI-MS in positive-ion mode to detect [M+Na]⁺ or [M+H]⁺ ions, ensuring no residual sulfonate intermediates remain .
Advanced Research Questions
Q. What mechanisms underlie the atypical reactivity of 4-thio-D-glucose derivatives in glycosylation reactions compared to oxygen analogues?
- Hypothesis-Driven Analysis : The C-S bond’s longer length (1.81 Å vs. 1.43 Å for C-O) reduces steric hindrance and alters transition-state stabilization. Computational studies (e.g., DFT) suggest sulfur’s lower electronegativity increases nucleophilicity at the anomeric center, favoring β-selectivity in glycosylations .
- Experimental Validation : Compare glycosylation kinetics (via stopped-flow NMR) between 4-thio and native glucose derivatives using thiophilic promoters like NIS/AgOTf. Monitor β/α ratios under varying temperatures .
Q. How can contradictory data on the bioactivity of 4-thio-D-glucose derivatives (e.g., cytotoxicity vs. metabolic inhibition) be resolved?
- Case Study : In high-glucose models (e.g., 25 mM D-glucose), 4-thio derivatives may exhibit conflicting effects:
- Cytotoxicity : Observed in cardiac progenitor cells (hCPCs) after 72-hour exposure, linked to downregulation of cell cycle proteins (cyclin D1, CDK4) via ROS overproduction .
- Protective Effects : In endothelial cells, insulin co-treatment reverses glucose-induced oxidative stress by restoring BH4 levels and MAPK phosphorylation .
Q. What advanced techniques are required to study the conformational dynamics of 4-S-β-D-glucopyranosyl-4-thio-D-glucose in solution?
- Methods :
Mutarotation Analysis : Use polarimetry to track optical rotation changes (e.g., α → β equilibrium) in aqueous solutions. Compare with O-glucose analogues to quantify sulfur’s impact on ring flexibility .
Molecular Dynamics (MD) Simulations : Parameterize the C-S bond using force fields (e.g., CHARMM) to model chair-to-boat transitions and glycosidic linkage flexibility .
NOESY NMR : Identify intra-ring nuclear Overhauser effects (NOEs) to map preferred chair conformations .
Q. Data Contradiction Analysis
Q. Why do studies report conflicting solubility profiles for 4-thio-D-glucose derivatives?
- Key Variables :
- Hydration State : Anhydrous vs. monohydrate forms (e.g., 4-O-β-D-galactopyranosyl-D-glucitol hydrate) exhibit divergent solubility in polar solvents .
- Crystallinity : Amorphous vs. crystalline preparations (confirmed via XRD) influence dissolution rates in PBS or DMSO .
- Mitigation : Pre-equilibrate compounds in controlled humidity chambers and report hydration status in experimental protocols .
Propriétés
IUPAC Name |
(2R,3R,4R,5R)-2,3,5,6-tetrahydroxy-4-[(2S,3R,4S,5S,6R)-3,4,5-trihydroxy-6-(hydroxymethyl)oxan-2-yl]sulfanylhexanal | |
---|---|---|
Source | PubChem | |
URL | https://pubchem.ncbi.nlm.nih.gov | |
Description | Data deposited in or computed by PubChem | |
InChI |
InChI=1S/C12H22O10S/c13-1-4(16)7(18)11(5(17)2-14)23-12-10(21)9(20)8(19)6(3-15)22-12/h1,4-12,14-21H,2-3H2/t4-,5+,6+,7+,8+,9-,10+,11+,12-/m0/s1 | |
Source | PubChem | |
URL | https://pubchem.ncbi.nlm.nih.gov | |
Description | Data deposited in or computed by PubChem | |
InChI Key |
VMEDEPBFFWJMMG-WELRSGGNSA-N | |
Source | PubChem | |
URL | https://pubchem.ncbi.nlm.nih.gov | |
Description | Data deposited in or computed by PubChem | |
Canonical SMILES |
C(C1C(C(C(C(O1)SC(C(CO)O)C(C(C=O)O)O)O)O)O)O | |
Source | PubChem | |
URL | https://pubchem.ncbi.nlm.nih.gov | |
Description | Data deposited in or computed by PubChem | |
Isomeric SMILES |
C([C@@H]1[C@H]([C@@H]([C@H]([C@@H](O1)S[C@H]([C@@H](CO)O)[C@@H]([C@H](C=O)O)O)O)O)O)O | |
Source | PubChem | |
URL | https://pubchem.ncbi.nlm.nih.gov | |
Description | Data deposited in or computed by PubChem | |
Molecular Formula |
C12H22O10S | |
Source | PubChem | |
URL | https://pubchem.ncbi.nlm.nih.gov | |
Description | Data deposited in or computed by PubChem | |
DSSTOX Substance ID |
DTXSID801001659 | |
Record name | 4-S-Hexopyranosyl-4-thiohexose | |
Source | EPA DSSTox | |
URL | https://comptox.epa.gov/dashboard/DTXSID801001659 | |
Description | DSSTox provides a high quality public chemistry resource for supporting improved predictive toxicology. | |
Molecular Weight |
358.36 g/mol | |
Source | PubChem | |
URL | https://pubchem.ncbi.nlm.nih.gov | |
Description | Data deposited in or computed by PubChem | |
CAS No. |
80951-92-4 | |
Record name | Thiocellobiose | |
Source | ChemIDplus | |
URL | https://pubchem.ncbi.nlm.nih.gov/substance/?source=chemidplus&sourceid=0080951924 | |
Description | ChemIDplus is a free, web search system that provides access to the structure and nomenclature authority files used for the identification of chemical substances cited in National Library of Medicine (NLM) databases, including the TOXNET system. | |
Record name | 4-S-Hexopyranosyl-4-thiohexose | |
Source | EPA DSSTox | |
URL | https://comptox.epa.gov/dashboard/DTXSID801001659 | |
Description | DSSTox provides a high quality public chemistry resource for supporting improved predictive toxicology. | |
Retrosynthesis Analysis
AI-Powered Synthesis Planning: Our tool employs the Template_relevance Pistachio, Template_relevance Bkms_metabolic, Template_relevance Pistachio_ringbreaker, Template_relevance Reaxys, Template_relevance Reaxys_biocatalysis model, leveraging a vast database of chemical reactions to predict feasible synthetic routes.
One-Step Synthesis Focus: Specifically designed for one-step synthesis, it provides concise and direct routes for your target compounds, streamlining the synthesis process.
Accurate Predictions: Utilizing the extensive PISTACHIO, BKMS_METABOLIC, PISTACHIO_RINGBREAKER, REAXYS, REAXYS_BIOCATALYSIS database, our tool offers high-accuracy predictions, reflecting the latest in chemical research and data.
Strategy Settings
Precursor scoring | Relevance Heuristic |
---|---|
Min. plausibility | 0.01 |
Model | Template_relevance |
Template Set | Pistachio/Bkms_metabolic/Pistachio_ringbreaker/Reaxys/Reaxys_biocatalysis |
Top-N result to add to graph | 6 |
Feasible Synthetic Routes
Avertissement et informations sur les produits de recherche in vitro
Veuillez noter que tous les articles et informations sur les produits présentés sur BenchChem sont destinés uniquement à des fins informatives. Les produits disponibles à l'achat sur BenchChem sont spécifiquement conçus pour des études in vitro, qui sont réalisées en dehors des organismes vivants. Les études in vitro, dérivées du terme latin "in verre", impliquent des expériences réalisées dans des environnements de laboratoire contrôlés à l'aide de cellules ou de tissus. Il est important de noter que ces produits ne sont pas classés comme médicaments et n'ont pas reçu l'approbation de la FDA pour la prévention, le traitement ou la guérison de toute condition médicale, affection ou maladie. Nous devons souligner que toute forme d'introduction corporelle de ces produits chez les humains ou les animaux est strictement interdite par la loi. Il est essentiel de respecter ces directives pour assurer la conformité aux normes légales et éthiques en matière de recherche et d'expérimentation.