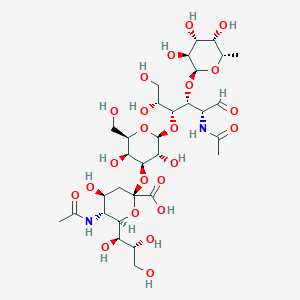
Sialyl-Lewis X
Vue d'ensemble
Description
Le chlorure de 2,3-diaminophénazinium, communément appelé DAPH, est un dérivé de la phénazine. Les composés de la phénazine sont connus pour leurs diverses activités biologiques et sont souvent utilisés en chimie médicinale et en science des matériaux. Le DAPH est particulièrement reconnu pour ses propriétés thérapeutiques potentielles, notamment ses activités antibactériennes et antivirales .
Applications De Recherche Scientifique
DAPH has a wide range of scientific research applications:
Chemistry: DAPH is used as a building block for synthesizing other phenazine derivatives.
Biology: DAPH exhibits antibacterial properties against clinical bacteria such as Streptococcus pneumoniae and Escherichia coli.
Industry: DAPH’s antibacterial properties make it a candidate for use in disinfectants and preservatives.
Analyse Biochimique
Biochemical Properties
Sialyl-Lewis X is involved in several biochemical reactions, primarily through its interactions with selectins, a family of cell adhesion molecules. It serves as a ligand for E-selectin, P-selectin, and L-selectin, facilitating the adhesion of leukocytes to endothelial cells during the inflammatory response . This interaction is mediated by the binding of this compound to the lectin domain of selectins, which is crucial for the rolling and tethering of leukocytes on the vascular endothelium . Additionally, this compound interacts with various glycosyltransferases and sialyltransferases, which are responsible for its biosynthesis and modification .
Cellular Effects
This compound influences various cellular processes, including cell signaling, gene expression, and cellular metabolism. In immune cells, it plays a pivotal role in the trafficking and homing of leukocytes to sites of inflammation . The binding of this compound to selectins triggers intracellular signaling pathways that enhance the expression of adhesion molecules and cytokines, promoting the inflammatory response . In cancer cells, overexpression of this compound is associated with increased metastatic potential, as it facilitates the adhesion and extravasation of tumor cells into distant tissues .
Molecular Mechanism
The molecular mechanism of this compound involves its specific binding interactions with selectins and other biomolecules. The tetrasaccharide structure of this compound allows it to fit into the binding pocket of selectins, forming multiple hydrogen bonds and van der Waals interactions . This binding induces conformational changes in the selectin molecules, enhancing their affinity for leukocytes and tumor cells . Additionally, this compound can modulate the activity of glycosyltransferases and sialyltransferases, influencing the glycosylation patterns of other glycoproteins and glycolipids .
Temporal Effects in Laboratory Settings
In laboratory settings, the effects of this compound can vary over time due to its stability and degradation. Studies have shown that this compound is relatively stable under physiological conditions, but its activity can be affected by enzymatic degradation and chemical modifications . Long-term exposure to this compound in in vitro and in vivo studies has demonstrated sustained effects on leukocyte adhesion and tumor cell metastasis, highlighting its potential as a therapeutic target .
Dosage Effects in Animal Models
The effects of this compound in animal models are dose-dependent. Low doses of this compound have been shown to enhance leukocyte adhesion and migration, while high doses can lead to adverse effects such as excessive inflammation and tissue damage . In cancer models, varying doses of this compound have been used to study its impact on tumor growth and metastasis, with higher doses generally correlating with increased metastatic potential .
Metabolic Pathways
This compound is involved in several metabolic pathways, including those related to glycosylation and sialylation. It is synthesized by the sequential action of glycosyltransferases and sialyltransferases, which add specific sugar residues to the growing oligosaccharide chain . This compound can also influence metabolic flux and metabolite levels by modulating the activity of these enzymes and altering the glycosylation patterns of other biomolecules .
Transport and Distribution
Within cells and tissues, this compound is transported and distributed through interactions with specific transporters and binding proteins. It is primarily localized on the cell surface, where it can interact with selectins and other cell adhesion molecules . The distribution of this compound can be influenced by its biosynthesis and degradation, as well as by the presence of other glycoproteins and glycolipids that share similar glycosylation patterns .
Subcellular Localization
This compound is predominantly localized on the plasma membrane, where it plays a key role in cell-cell interactions and signaling . It can also be found in the Golgi apparatus, where it is synthesized and modified by glycosyltransferases and sialyltransferases . The subcellular localization of this compound is regulated by targeting signals and post-translational modifications that direct it to specific compartments and organelles .
Méthodes De Préparation
Voies de synthèse et conditions de réaction
Le DAPH peut être synthétisé par oxydation catalytique directe de l'o-phénylène diamine avec un complexe de fer(III), spécifiquement [Fe(1,10-phénanthroline)2Cl2]NO3, dans l'éthanol en conditions aérobies . La réaction implique les étapes suivantes :
- Dissoudre l'o-phénylène diamine dans l'éthanol.
- Ajouter le complexe de fer(III) à la solution.
- Laisser la réaction se dérouler en conditions aérobies.
- Isoler le produit par cristallisation.
La structure cristalline et la complexité moléculaire du DAPH sont confirmées à l'aide de diverses méthodes spectroscopiques et d'une analyse structurale par diffraction des rayons X sur monocristal .
Méthodes de production industrielle
Bien que les méthodes de production industrielle spécifiques du DAPH ne soient pas largement documentées, l'approche générale consiste à mettre à l'échelle le processus de synthèse en laboratoire. Cela comprend l'optimisation des conditions de réaction, telles que la température, la pression et le choix du solvant, pour assurer un rendement et une pureté élevés. La production industrielle peut également impliquer des réacteurs à écoulement continu pour améliorer l'efficacité et l'extensibilité.
Analyse Des Réactions Chimiques
Types de réactions
Le DAPH subit plusieurs types de réactions chimiques, notamment :
Oxydation : Le DAPH peut être oxydé pour former divers dérivés de la phénazine.
Réduction : Les réactions de réduction peuvent convertir le DAPH en ses formes réduites, qui peuvent présenter des activités biologiques différentes.
Substitution : Le DAPH peut participer à des réactions de substitution, où les groupes fonctionnels du cycle phénazinique sont remplacés par d'autres groupes.
Réactifs et conditions courants
Oxydation : Les oxydants courants comprennent le peroxyde d'hydrogène et le permanganate de potassium.
Réduction : Des agents réducteurs tels que le borohydrure de sodium ou l'hydrure d'aluminium et de lithium sont utilisés.
Substitution : Les réactions de substitution impliquent souvent des nucléophiles comme les amines ou les thiols en conditions basiques.
Principaux produits
Les principaux produits formés à partir de ces réactions dépendent des réactifs et des conditions spécifiques utilisés. Par exemple, l'oxydation du DAPH peut produire divers dérivés de la phénazine présentant des activités biologiques potentielles .
Applications de la recherche scientifique
Le DAPH a un large éventail d'applications de recherche scientifique :
Chimie : Le DAPH est utilisé comme bloc de construction pour la synthèse d'autres dérivés de la phénazine.
Biologie : Le DAPH présente des propriétés antibactériennes contre des bactéries cliniques telles que Streptococcus pneumoniae et Escherichia coli.
Industrie : Les propriétés antibactériennes du DAPH en font un candidat potentiel pour une utilisation dans les désinfectants et les conservateurs.
Mécanisme d'action
Le DAPH exerce ses effets par plusieurs mécanismes :
Inhibition des enzymes : Le DAPH peut inhiber des enzymes telles que la BACE1, qui est impliquée dans la formation de plaques d'amyloïde-bêta dans la maladie d'Alzheimer.
Activité antioxydante : Le DAPH active la voie Nrf2/HO-1, ce qui contribue à réduire le stress oxydatif et l'inflammation.
Activité antibactérienne : Le DAPH perturbe les membranes cellulaires bactériennes, ce qui conduit à la mort cellulaire.
Mécanisme D'action
DAPH exerts its effects through several mechanisms:
Inhibition of Enzymes: DAPH can inhibit enzymes such as BACE1, which is involved in the formation of amyloid-beta plaques in Alzheimer’s disease.
Antioxidant Activity: DAPH activates the Nrf2/HO-1 pathway, which helps reduce oxidative stress and inflammation.
Antibacterial Activity: DAPH disrupts bacterial cell membranes, leading to cell death.
Comparaison Avec Des Composés Similaires
Le DAPH peut être comparé à d'autres dérivés de la phénazine, tels que :
Acide phénazine-1-carboxylique : Connu pour ses propriétés antifongiques.
Pyocyanine : Présente des activités antibactériennes et cytotoxiques.
Acide phénazine-1,6-dicarboxylique : Utilisé dans la synthèse de divers composés organiques.
Unicité
Le DAPH est unique en raison de ses activités antibactériennes et antivirales doubles, ainsi que de ses applications thérapeutiques potentielles dans les maladies neurodégénératives . Sa capacité à inhiber la formation de fibrilles d'amyloïde-bêta le distingue des autres dérivés de la phénazine.
Propriétés
IUPAC Name |
(2S,4S,5R,6R)-5-acetamido-2-[(2S,3R,4S,5S,6R)-2-[(2R,3R,4R,5R)-5-acetamido-1,2-dihydroxy-6-oxo-4-[(2S,3S,4R,5S,6S)-3,4,5-trihydroxy-6-methyloxan-2-yl]oxyhexan-3-yl]oxy-3,5-dihydroxy-6-(hydroxymethyl)oxan-4-yl]oxy-4-hydroxy-6-[(1R,2R)-1,2,3-trihydroxypropyl]oxane-2-carboxylic acid | |
---|---|---|
Source | PubChem | |
URL | https://pubchem.ncbi.nlm.nih.gov | |
Description | Data deposited in or computed by PubChem | |
InChI |
InChI=1S/C31H52N2O23/c1-9-18(43)21(46)22(47)28(51-9)53-24(12(5-34)32-10(2)38)25(15(42)7-36)54-29-23(48)27(20(45)16(8-37)52-29)56-31(30(49)50)4-13(40)17(33-11(3)39)26(55-31)19(44)14(41)6-35/h5,9,12-29,35-37,40-48H,4,6-8H2,1-3H3,(H,32,38)(H,33,39)(H,49,50)/t9-,12-,13-,14+,15+,16+,17+,18+,19+,20-,21+,22-,23+,24+,25+,26+,27-,28-,29-,31-/m0/s1 | |
Source | PubChem | |
URL | https://pubchem.ncbi.nlm.nih.gov | |
Description | Data deposited in or computed by PubChem | |
InChI Key |
LAQPKDLYOBZWBT-NYLDSJSYSA-N | |
Source | PubChem | |
URL | https://pubchem.ncbi.nlm.nih.gov | |
Description | Data deposited in or computed by PubChem | |
Canonical SMILES |
CC1C(C(C(C(O1)OC(C(C=O)NC(=O)C)C(C(CO)O)OC2C(C(C(C(O2)CO)O)OC3(CC(C(C(O3)C(C(CO)O)O)NC(=O)C)O)C(=O)O)O)O)O)O | |
Source | PubChem | |
URL | https://pubchem.ncbi.nlm.nih.gov | |
Description | Data deposited in or computed by PubChem | |
Isomeric SMILES |
C[C@H]1[C@H]([C@H]([C@@H]([C@@H](O1)O[C@H]([C@H](C=O)NC(=O)C)[C@@H]([C@@H](CO)O)O[C@H]2[C@@H]([C@H]([C@H]([C@H](O2)CO)O)O[C@@]3(C[C@@H]([C@H]([C@@H](O3)[C@@H]([C@@H](CO)O)O)NC(=O)C)O)C(=O)O)O)O)O)O | |
Source | PubChem | |
URL | https://pubchem.ncbi.nlm.nih.gov | |
Description | Data deposited in or computed by PubChem | |
Molecular Formula |
C31H52N2O23 | |
Source | PubChem | |
URL | https://pubchem.ncbi.nlm.nih.gov | |
Description | Data deposited in or computed by PubChem | |
DSSTOX Substance ID |
DTXSID60913167 | |
Record name | 3′-Sialyl-Lewis X | |
Source | EPA DSSTox | |
URL | https://comptox.epa.gov/dashboard/DTXSID60913167 | |
Description | DSSTox provides a high quality public chemistry resource for supporting improved predictive toxicology. | |
Molecular Weight |
820.7 g/mol | |
Source | PubChem | |
URL | https://pubchem.ncbi.nlm.nih.gov | |
Description | Data deposited in or computed by PubChem | |
Physical Description |
Solid | |
Record name | Sialyl-Lewis X | |
Source | Human Metabolome Database (HMDB) | |
URL | http://www.hmdb.ca/metabolites/HMDB0006565 | |
Description | The Human Metabolome Database (HMDB) is a freely available electronic database containing detailed information about small molecule metabolites found in the human body. | |
Explanation | HMDB is offered to the public as a freely available resource. Use and re-distribution of the data, in whole or in part, for commercial purposes requires explicit permission of the authors and explicit acknowledgment of the source material (HMDB) and the original publication (see the HMDB citing page). We ask that users who download significant portions of the database cite the HMDB paper in any resulting publications. | |
CAS No. |
98603-84-0 | |
Record name | Sialyl-Lewis X | |
Source | CAS Common Chemistry | |
URL | https://commonchemistry.cas.org/detail?cas_rn=98603-84-0 | |
Description | CAS Common Chemistry is an open community resource for accessing chemical information. Nearly 500,000 chemical substances from CAS REGISTRY cover areas of community interest, including common and frequently regulated chemicals, and those relevant to high school and undergraduate chemistry classes. This chemical information, curated by our expert scientists, is provided in alignment with our mission as a division of the American Chemical Society. | |
Explanation | The data from CAS Common Chemistry is provided under a CC-BY-NC 4.0 license, unless otherwise stated. | |
Record name | Sialyl lewis X | |
Source | ChemIDplus | |
URL | https://pubchem.ncbi.nlm.nih.gov/substance/?source=chemidplus&sourceid=0098603840 | |
Description | ChemIDplus is a free, web search system that provides access to the structure and nomenclature authority files used for the identification of chemical substances cited in National Library of Medicine (NLM) databases, including the TOXNET system. | |
Record name | 3′-Sialyl-Lewis X | |
Source | EPA DSSTox | |
URL | https://comptox.epa.gov/dashboard/DTXSID60913167 | |
Description | DSSTox provides a high quality public chemistry resource for supporting improved predictive toxicology. | |
Record name | SIALYL LEWIS X | |
Source | FDA Global Substance Registration System (GSRS) | |
URL | https://gsrs.ncats.nih.gov/ginas/app/beta/substances/0PS35WG8U3 | |
Description | The FDA Global Substance Registration System (GSRS) enables the efficient and accurate exchange of information on what substances are in regulated products. Instead of relying on names, which vary across regulatory domains, countries, and regions, the GSRS knowledge base makes it possible for substances to be defined by standardized, scientific descriptions. | |
Explanation | Unless otherwise noted, the contents of the FDA website (www.fda.gov), both text and graphics, are not copyrighted. They are in the public domain and may be republished, reprinted and otherwise used freely by anyone without the need to obtain permission from FDA. Credit to the U.S. Food and Drug Administration as the source is appreciated but not required. | |
Record name | Sialyl-Lewis X | |
Source | Human Metabolome Database (HMDB) | |
URL | http://www.hmdb.ca/metabolites/HMDB0006565 | |
Description | The Human Metabolome Database (HMDB) is a freely available electronic database containing detailed information about small molecule metabolites found in the human body. | |
Explanation | HMDB is offered to the public as a freely available resource. Use and re-distribution of the data, in whole or in part, for commercial purposes requires explicit permission of the authors and explicit acknowledgment of the source material (HMDB) and the original publication (see the HMDB citing page). We ask that users who download significant portions of the database cite the HMDB paper in any resulting publications. | |
Retrosynthesis Analysis
AI-Powered Synthesis Planning: Our tool employs the Template_relevance Pistachio, Template_relevance Bkms_metabolic, Template_relevance Pistachio_ringbreaker, Template_relevance Reaxys, Template_relevance Reaxys_biocatalysis model, leveraging a vast database of chemical reactions to predict feasible synthetic routes.
One-Step Synthesis Focus: Specifically designed for one-step synthesis, it provides concise and direct routes for your target compounds, streamlining the synthesis process.
Accurate Predictions: Utilizing the extensive PISTACHIO, BKMS_METABOLIC, PISTACHIO_RINGBREAKER, REAXYS, REAXYS_BIOCATALYSIS database, our tool offers high-accuracy predictions, reflecting the latest in chemical research and data.
Strategy Settings
Precursor scoring | Relevance Heuristic |
---|---|
Min. plausibility | 0.01 |
Model | Template_relevance |
Template Set | Pistachio/Bkms_metabolic/Pistachio_ringbreaker/Reaxys/Reaxys_biocatalysis |
Top-N result to add to graph | 6 |
Feasible Synthetic Routes
Avertissement et informations sur les produits de recherche in vitro
Veuillez noter que tous les articles et informations sur les produits présentés sur BenchChem sont destinés uniquement à des fins informatives. Les produits disponibles à l'achat sur BenchChem sont spécifiquement conçus pour des études in vitro, qui sont réalisées en dehors des organismes vivants. Les études in vitro, dérivées du terme latin "in verre", impliquent des expériences réalisées dans des environnements de laboratoire contrôlés à l'aide de cellules ou de tissus. Il est important de noter que ces produits ne sont pas classés comme médicaments et n'ont pas reçu l'approbation de la FDA pour la prévention, le traitement ou la guérison de toute condition médicale, affection ou maladie. Nous devons souligner que toute forme d'introduction corporelle de ces produits chez les humains ou les animaux est strictement interdite par la loi. Il est essentiel de respecter ces directives pour assurer la conformité aux normes légales et éthiques en matière de recherche et d'expérimentation.