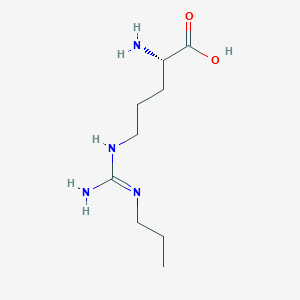
N-omega-Propyl-L-arginine
Vue d'ensemble
Description
Ces composés contiennent de l'arginine ou un dérivé de celle-ci résultant de la réaction de l'arginine au niveau du groupe amino ou du groupe carboxy, ou du remplacement de tout hydrogène de la glycine par un hétéroatome . La N-oméga-propyl-L-arginine est un inhibiteur puissant, compétitif et hautement sélectif de la synthase d'oxyde nitrique neuronale .
Méthodes De Préparation
Voies de synthèse et conditions de réaction
La N-oméga-propyl-L-arginine peut être synthétisée par une série de réactions chimiques impliquant la modification de la molécule d'arginineCela peut être réalisé par diverses réactions chimiques, notamment des réactions d'alkylation et d'amidation .
Méthodes de production industrielle
La production industrielle de N-oméga-propyl-L-arginine implique une synthèse à grande échelle utilisant des conditions de réaction optimisées pour garantir un rendement et une pureté élevés. Le processus peut impliquer l'utilisation d'équipements et de réactifs spécialisés pour faciliter les étapes de réaction et de purification. Le composé est généralement produit sous forme solide et stocké dans des conditions contrôlées pour maintenir sa stabilité .
Analyse Des Réactions Chimiques
Types de réactions
La N-oméga-propyl-L-arginine subit diverses réactions chimiques, notamment :
Oxydation : Le composé peut subir des réactions d'oxydation, conduisant à la formation de dérivés oxydés.
Réduction : Les réactions de réduction peuvent convertir le composé en formes réduites présentant des propriétés chimiques différentes.
Substitution : Les réactions de substitution impliquent le remplacement de groupes fonctionnels spécifiques au sein de la molécule par d'autres groupes.
Réactifs et conditions courants
Les réactifs courants utilisés dans les réactions de N-oméga-propyl-L-arginine comprennent des agents oxydants, des agents réducteurs et divers catalyseurs. Les conditions de réaction, telles que la température, la pression et le solvant, sont optimisées pour obtenir les transformations chimiques souhaitées .
Principaux produits formés
Les principaux produits formés à partir des réactions de N-oméga-propyl-L-arginine dépendent du type de réaction spécifique et des réactifs utilisés. Par exemple, les réactions d'oxydation peuvent produire des dérivés oxydés, tandis que les réactions de substitution peuvent produire des analogues substitués du composé .
Applications de la recherche scientifique
La N-oméga-propyl-L-arginine présente un large éventail d'applications de recherche scientifique, notamment :
Chimie : Le composé est utilisé comme réactif dans diverses réactions chimiques et études pour explorer sa réactivité et ses propriétés.
Biologie : En recherche biologique, la N-oméga-propyl-L-arginine est utilisée pour étudier le rôle de la synthase d'oxyde nitrique dans les processus cellulaires et les voies de signalisation.
Médecine : Le composé est étudié pour ses applications thérapeutiques potentielles, en particulier comme inhibiteur de la synthase d'oxyde nitrique neuronale, qui est impliquée dans divers troubles neurologiques.
Industrie : La N-oméga-propyl-L-arginine est utilisée dans le développement de nouveaux matériaux et produits aux propriétés chimiques spécifiques
Mécanisme d'action
La N-oméga-propyl-L-arginine exerce ses effets en inhibant la synthase d'oxyde nitrique neuronale. Cette enzyme est responsable de la production d'oxyde nitrique, une molécule de signalisation impliquée dans divers processus physiologiques. En inhibant cette enzyme, la N-oméga-propyl-L-arginine réduit la production d'oxyde nitrique, modulant ainsi ses effets sur la signalisation cellulaire et la fonction .
Applications De Recherche Scientifique
Pharmacological Properties
NPA is recognized for its ability to selectively inhibit nNOS, which plays a critical role in the production of nitric oxide (NO), a crucial signaling molecule in the nervous system. The compound demonstrates competitive inhibition, making it a valuable tool in studying the physiological and pathological roles of NO in various biological systems.
Neurological Studies
NPA has been extensively used in neurological research to understand the role of NO in neurodegenerative diseases and psychiatric disorders. For instance, studies have shown that NPA can block the effects of phencyclidine (PCP) on locomotor activity and prepulse inhibition in mice, suggesting its potential use in exploring schizophrenia models .
Cardiovascular Research
In cardiovascular studies, NPA has been utilized to investigate its protective effects against reperfusion injury in skeletal muscle. Research indicates that NPA can mitigate oxidative stress and improve recovery following ischemic events, highlighting its potential therapeutic applications in heart disease .
Cancer Research
The compound's role in modulating inflammation through NO pathways has implications for cancer research. By inhibiting nNOS, NPA may influence tumor microenvironments and enhance the efficacy of certain chemotherapeutic agents .
Effects on Ischemic Injury
A notable study by Gowda et al. (2004) demonstrated that NPA significantly reduced tissue damage and improved functional recovery in models of skeletal muscle ischemia. The findings suggest that targeting nNOS with NPA could be a promising strategy for enhancing recovery post-injury .
Impact on Neurobehavioral Outcomes
Klamer et al. (2004) investigated the effects of NPA on neurobehavioral outcomes related to PCP-induced behaviors in mice. The study concluded that NPA effectively normalized locomotor activity disrupted by PCP, supporting its potential use as a therapeutic agent in treating psychotic disorders .
Mécanisme D'action
N-Omega-Propyl-L-Arginine exerts its effects by inhibiting neuronal nitric oxide synthase. This enzyme is responsible for the production of nitric oxide, a signaling molecule involved in various physiological processes. By inhibiting this enzyme, this compound reduces the production of nitric oxide, thereby modulating its effects on cellular signaling and function .
Comparaison Avec Des Composés Similaires
Composés similaires
Les composés similaires à la N-oméga-propyl-L-arginine comprennent d'autres dérivés de l'arginine et des inhibiteurs de la synthase d'oxyde nitrique, tels que :
- N-oméga-nitro-L-arginine
- N-oméga-méthyl-L-arginine
- N-oméga-hydroxy-L-arginine .
Unicité
La N-oméga-propyl-L-arginine est unique en raison de sa forte sélectivité et de sa puissance en tant qu'inhibiteur de la synthase d'oxyde nitrique neuronale. Cette sélectivité en fait un outil précieux dans la recherche et les applications thérapeutiques potentielles, car elle permet une modulation ciblée de la production d'oxyde nitrique sans affecter les autres isoformes de l'enzyme .
Activité Biologique
N-omega-Propyl-L-arginine (NPA) is a selective inhibitor of neuronal nitric oxide synthase (nNOS), which plays a significant role in various physiological and pathological processes. This article provides an overview of the biological activity of NPA, including its mechanisms, effects on various conditions, and relevant research findings.
NPA selectively inhibits nNOS with a potency that is significantly higher than its effects on inducible nitric oxide synthase (iNOS) and endothelial nitric oxide synthase (eNOS). Specifically, it has been reported to be 3158-fold more selective for nNOS compared to iNOS and 149-fold compared to eNOS . The inhibition mechanism involves reversible binding at the arginine substrate site of the enzyme, which prevents the conversion of L-arginine to nitric oxide (NO) and L-citrulline.
Biological Effects
-
Neuroprotective Effects :
- NPA has shown promising results in models of epilepsy. In studies involving kainic acid-induced status epilepticus in mice, NPA treatment significantly reduced seizure severity and duration, as well as the frequency of epileptiform spikes. It also decreased neuronal activity and gliosis, suggesting its potential as a neuroprotective agent in epilepsy management .
- Cardiovascular Effects :
- Role in Pain Management :
Table 1: Summary of Key Studies on this compound
Propriétés
IUPAC Name |
(2S)-2-amino-5-[(N'-propylcarbamimidoyl)amino]pentanoic acid | |
---|---|---|
Source | PubChem | |
URL | https://pubchem.ncbi.nlm.nih.gov | |
Description | Data deposited in or computed by PubChem | |
InChI |
InChI=1S/C9H20N4O2/c1-2-5-12-9(11)13-6-3-4-7(10)8(14)15/h7H,2-6,10H2,1H3,(H,14,15)(H3,11,12,13)/t7-/m0/s1 | |
Source | PubChem | |
URL | https://pubchem.ncbi.nlm.nih.gov | |
Description | Data deposited in or computed by PubChem | |
InChI Key |
AOMXURITGZJPKB-ZETCQYMHSA-N | |
Source | PubChem | |
URL | https://pubchem.ncbi.nlm.nih.gov | |
Description | Data deposited in or computed by PubChem | |
Canonical SMILES |
CCCN=C(N)NCCCC(C(=O)O)N | |
Source | PubChem | |
URL | https://pubchem.ncbi.nlm.nih.gov | |
Description | Data deposited in or computed by PubChem | |
Isomeric SMILES |
CCCN=C(N)NCCC[C@@H](C(=O)O)N | |
Source | PubChem | |
URL | https://pubchem.ncbi.nlm.nih.gov | |
Description | Data deposited in or computed by PubChem | |
Molecular Formula |
C9H20N4O2 | |
Source | PubChem | |
URL | https://pubchem.ncbi.nlm.nih.gov | |
Description | Data deposited in or computed by PubChem | |
DSSTOX Substance ID |
DTXSID30332245 | |
Record name | (2S)-2-Amino-5-(N'-propylcarbamimidamido)pentanoic acid | |
Source | EPA DSSTox | |
URL | https://comptox.epa.gov/dashboard/DTXSID30332245 | |
Description | DSSTox provides a high quality public chemistry resource for supporting improved predictive toxicology. | |
Molecular Weight |
216.28 g/mol | |
Source | PubChem | |
URL | https://pubchem.ncbi.nlm.nih.gov | |
Description | Data deposited in or computed by PubChem | |
CAS No. |
137361-05-8 | |
Record name | Nω-Propyl-L-arginine | |
Source | CAS Common Chemistry | |
URL | https://commonchemistry.cas.org/detail?cas_rn=137361-05-8 | |
Description | CAS Common Chemistry is an open community resource for accessing chemical information. Nearly 500,000 chemical substances from CAS REGISTRY cover areas of community interest, including common and frequently regulated chemicals, and those relevant to high school and undergraduate chemistry classes. This chemical information, curated by our expert scientists, is provided in alignment with our mission as a division of the American Chemical Society. | |
Explanation | The data from CAS Common Chemistry is provided under a CC-BY-NC 4.0 license, unless otherwise stated. | |
Record name | (2S)-2-Amino-5-(N'-propylcarbamimidamido)pentanoic acid | |
Source | EPA DSSTox | |
URL | https://comptox.epa.gov/dashboard/DTXSID30332245 | |
Description | DSSTox provides a high quality public chemistry resource for supporting improved predictive toxicology. | |
Record name | Nω-Propyl-L-arginine | |
Source | FDA Global Substance Registration System (GSRS) | |
URL | https://gsrs.ncats.nih.gov/ginas/app/beta/substances/G7F9U4R2AC | |
Description | The FDA Global Substance Registration System (GSRS) enables the efficient and accurate exchange of information on what substances are in regulated products. Instead of relying on names, which vary across regulatory domains, countries, and regions, the GSRS knowledge base makes it possible for substances to be defined by standardized, scientific descriptions. | |
Explanation | Unless otherwise noted, the contents of the FDA website (www.fda.gov), both text and graphics, are not copyrighted. They are in the public domain and may be republished, reprinted and otherwise used freely by anyone without the need to obtain permission from FDA. Credit to the U.S. Food and Drug Administration as the source is appreciated but not required. | |
Retrosynthesis Analysis
AI-Powered Synthesis Planning: Our tool employs the Template_relevance Pistachio, Template_relevance Bkms_metabolic, Template_relevance Pistachio_ringbreaker, Template_relevance Reaxys, Template_relevance Reaxys_biocatalysis model, leveraging a vast database of chemical reactions to predict feasible synthetic routes.
One-Step Synthesis Focus: Specifically designed for one-step synthesis, it provides concise and direct routes for your target compounds, streamlining the synthesis process.
Accurate Predictions: Utilizing the extensive PISTACHIO, BKMS_METABOLIC, PISTACHIO_RINGBREAKER, REAXYS, REAXYS_BIOCATALYSIS database, our tool offers high-accuracy predictions, reflecting the latest in chemical research and data.
Strategy Settings
Precursor scoring | Relevance Heuristic |
---|---|
Min. plausibility | 0.01 |
Model | Template_relevance |
Template Set | Pistachio/Bkms_metabolic/Pistachio_ringbreaker/Reaxys/Reaxys_biocatalysis |
Top-N result to add to graph | 6 |
Feasible Synthetic Routes
Q1: How does N-omega-Propyl-L-arginine interact with its target and what are the downstream effects?
A1: this compound (NPLA) acts as a selective inhibitor of neuronal nitric oxide synthase (nNOS). [, , , ] This selectivity arises from its ability to bind to nNOS in the presence of calcium ions (Ca2+) and calmodulin (CaM), leading to a time- and concentration-dependent suppression of nitric oxide (NO) formation. [] This suppression is reversible and occurs through a first-order kinetic process. [] While NPLA can bind to nNOS rapidly, the subsequent conformational change induced by Ca2+/CaM binding slows down its dissociation, leading to prolonged inhibition. [] By inhibiting nNOS, NPLA reduces the production of NO, a key signaling molecule involved in various physiological processes, including vasodilation. [, , , , , ]
Q2: What insights do we have regarding the selectivity of this compound for nNOS?
A2: While this compound (NPLA) is widely recognized as a selective inhibitor of neuronal nitric oxide synthase (nNOS), some studies have raised questions about its absolute selectivity, particularly in in vivo settings. [] Research investigating the effects of NPLA on reperfusion injury in rat skeletal muscle found that NPLA treatment influenced both nNOS and eNOS mRNA and protein levels. [] This observation suggests that the in vivo effects of NPLA might not be solely attributed to nNOS inhibition and could involve interactions with other NOS isoforms. [] Therefore, further research is needed to fully elucidate the in vivo mechanisms of action of NPLA and confirm its selectivity profile across different experimental models.
Avertissement et informations sur les produits de recherche in vitro
Veuillez noter que tous les articles et informations sur les produits présentés sur BenchChem sont destinés uniquement à des fins informatives. Les produits disponibles à l'achat sur BenchChem sont spécifiquement conçus pour des études in vitro, qui sont réalisées en dehors des organismes vivants. Les études in vitro, dérivées du terme latin "in verre", impliquent des expériences réalisées dans des environnements de laboratoire contrôlés à l'aide de cellules ou de tissus. Il est important de noter que ces produits ne sont pas classés comme médicaments et n'ont pas reçu l'approbation de la FDA pour la prévention, le traitement ou la guérison de toute condition médicale, affection ou maladie. Nous devons souligner que toute forme d'introduction corporelle de ces produits chez les humains ou les animaux est strictement interdite par la loi. Il est essentiel de respecter ces directives pour assurer la conformité aux normes légales et éthiques en matière de recherche et d'expérimentation.