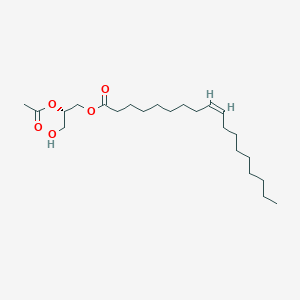
1-Oleoyl-2-acetyl-sn-glycerol
Vue d'ensemble
Description
1-Oleoyl-2-acetyl-sn-glycerol (OAG) is a synthetic, cell permeable diacylglycerol analog . It is often used as an activator of calcium-dependent protein kinase C (PKC) . The empirical formula is C23H42O5 .
Molecular Structure Analysis
The molecular weight of 1-Oleoyl-2-acetyl-sn-glycerol is 398.58 . The molecular formula is C23H42O5 .Chemical Reactions Analysis
1-Oleoyl-2-acetyl-sn-glycerol is known to activate calcium-dependent protein kinase C (PKC) and induce the production of superoxide . It is also metabolized to its corresponding phosphatidic acid (1-oleoyl-2-acetyl-3-phosphoglycerol), most likely through the action of a diglycerol kinase .Physical And Chemical Properties Analysis
1-Oleoyl-2-acetyl-sn-glycerol is an oil . It is soluble in ethanol and DMSO . The boiling point is predicted to be 490.0±15.0 °C and the density is predicted to be 0.980±0.06 g/cm3 .Applications De Recherche Scientifique
Protein Kinase C (PKC) Activation
OAG is a synthetic, cell permeable diacylglycerol analog . It is often used as an activator of calcium-dependent protein kinase C (PKC) . PKC is a family of protein kinase enzymes that are involved in controlling the function of other proteins through the phosphorylation of hydroxyl groups of serine and threonine amino acid residues .
Platelet Activation
OAG has been found to activate PKC in platelets . This activation results in the phosphorylation of a 40 kDa protein . This process is crucial for platelet activation, which plays a key role in blood clotting .
Actin Nucleation Sites Formation
OAG stimulates the formation of actin nucleation sites . Actin is a protein that forms (together with myosin) the contractile filaments of muscle cells, and is also involved in motion in other types of cells .
Phosphorylation of pp60c-src
OAG has been shown to stimulate the phosphorylation of pp60c-src in EBV transformed B-cells . pp60c-src is a protein that in humans is encoded by the SRC gene. It belongs to the src family of genes, which is a group of proto-oncogenes that code for tyrosine kinases .
Metabolization to Phosphatidic Acid
OAG is metabolized to its corresponding phosphatidic acid (1-oleoyl-2-acetyl-3-phosphoglycerol), most likely through the action of a diglycerol kinase . Phosphatidic acid is a phospholipid, a type of lipid molecule that is the main component of the cell membrane .
Research in Lipid Biochemistry
OAG is used in research related to lipid biochemistry . Lipids are a group of naturally occurring molecules that include fats, waxes, sterols, fat-soluble vitamins (such as vitamins A, D, E, and K), monoglycerides, diglycerides, triglycerides, phospholipids, and others .
Mécanisme D'action
Target of Action
The primary target of 1-Oleoyl-2-acetyl-sn-glycerol is the calcium-dependent protein kinase C (PKC) . PKC is a family of protein kinases that play crucial roles in several cellular processes, including cell differentiation, proliferation, and immune response .
Mode of Action
1-Oleoyl-2-acetyl-sn-glycerol is a synthetic, cell-permeable diacylglycerol analog . It interacts with its target, PKC, by activating it . This activation results in the phosphorylation of a 40 kDa protein .
Biochemical Pathways
The activation of PKC by 1-Oleoyl-2-acetyl-sn-glycerol affects several biochemical pathways. One of the key pathways is the second messenger system, specifically the diacylglycerol (DAG) pathway . The compound is metabolized to its corresponding phosphatidic acid (1-oleoyl-2-acetyl-3-phosphoglycerol), most likely through the action of a diglycerol kinase .
Pharmacokinetics
It is known that the compound is cell-permeable , which suggests it can readily cross cell membranes and reach its target site of action. This property could potentially enhance its bioavailability.
Result of Action
The activation of PKC by 1-Oleoyl-2-acetyl-sn-glycerol leads to the phosphorylation of a 40 kDa protein . This phosphorylation event can trigger a cascade of downstream cellular processes, potentially influencing cell differentiation, proliferation, and immune response .
Safety and Hazards
Propriétés
IUPAC Name |
[(2S)-2-acetyloxy-3-hydroxypropyl] (Z)-octadec-9-enoate | |
---|---|---|
Source | PubChem | |
URL | https://pubchem.ncbi.nlm.nih.gov | |
Description | Data deposited in or computed by PubChem | |
InChI |
InChI=1S/C23H42O5/c1-3-4-5-6-7-8-9-10-11-12-13-14-15-16-17-18-23(26)27-20-22(19-24)28-21(2)25/h10-11,22,24H,3-9,12-20H2,1-2H3/b11-10-/t22-/m0/s1 | |
Source | PubChem | |
URL | https://pubchem.ncbi.nlm.nih.gov | |
Description | Data deposited in or computed by PubChem | |
InChI Key |
PWTCCMJTPHCGMS-YRBAHSOBSA-N | |
Source | PubChem | |
URL | https://pubchem.ncbi.nlm.nih.gov | |
Description | Data deposited in or computed by PubChem | |
Canonical SMILES |
CCCCCCCCC=CCCCCCCCC(=O)OCC(CO)OC(=O)C | |
Source | PubChem | |
URL | https://pubchem.ncbi.nlm.nih.gov | |
Description | Data deposited in or computed by PubChem | |
Isomeric SMILES |
CCCCCCCC/C=C\CCCCCCCC(=O)OC[C@H](CO)OC(=O)C | |
Source | PubChem | |
URL | https://pubchem.ncbi.nlm.nih.gov | |
Description | Data deposited in or computed by PubChem | |
Molecular Formula |
C23H42O5 | |
Source | PubChem | |
URL | https://pubchem.ncbi.nlm.nih.gov | |
Description | Data deposited in or computed by PubChem | |
Molecular Weight |
398.6 g/mol | |
Source | PubChem | |
URL | https://pubchem.ncbi.nlm.nih.gov | |
Description | Data deposited in or computed by PubChem | |
Product Name |
1-Oleoyl-2-acetyl-sn-glycerol | |
CAS RN |
86390-77-4 | |
Record name | 1-Oleoyl-2-acetylglycerol | |
Source | ChemIDplus | |
URL | https://pubchem.ncbi.nlm.nih.gov/substance/?source=chemidplus&sourceid=0086390774 | |
Description | ChemIDplus is a free, web search system that provides access to the structure and nomenclature authority files used for the identification of chemical substances cited in National Library of Medicine (NLM) databases, including the TOXNET system. | |
Retrosynthesis Analysis
AI-Powered Synthesis Planning: Our tool employs the Template_relevance Pistachio, Template_relevance Bkms_metabolic, Template_relevance Pistachio_ringbreaker, Template_relevance Reaxys, Template_relevance Reaxys_biocatalysis model, leveraging a vast database of chemical reactions to predict feasible synthetic routes.
One-Step Synthesis Focus: Specifically designed for one-step synthesis, it provides concise and direct routes for your target compounds, streamlining the synthesis process.
Accurate Predictions: Utilizing the extensive PISTACHIO, BKMS_METABOLIC, PISTACHIO_RINGBREAKER, REAXYS, REAXYS_BIOCATALYSIS database, our tool offers high-accuracy predictions, reflecting the latest in chemical research and data.
Strategy Settings
Precursor scoring | Relevance Heuristic |
---|---|
Min. plausibility | 0.01 |
Model | Template_relevance |
Template Set | Pistachio/Bkms_metabolic/Pistachio_ringbreaker/Reaxys/Reaxys_biocatalysis |
Top-N result to add to graph | 6 |
Feasible Synthetic Routes
Avertissement et informations sur les produits de recherche in vitro
Veuillez noter que tous les articles et informations sur les produits présentés sur BenchChem sont destinés uniquement à des fins informatives. Les produits disponibles à l'achat sur BenchChem sont spécifiquement conçus pour des études in vitro, qui sont réalisées en dehors des organismes vivants. Les études in vitro, dérivées du terme latin "in verre", impliquent des expériences réalisées dans des environnements de laboratoire contrôlés à l'aide de cellules ou de tissus. Il est important de noter que ces produits ne sont pas classés comme médicaments et n'ont pas reçu l'approbation de la FDA pour la prévention, le traitement ou la guérison de toute condition médicale, affection ou maladie. Nous devons souligner que toute forme d'introduction corporelle de ces produits chez les humains ou les animaux est strictement interdite par la loi. Il est essentiel de respecter ces directives pour assurer la conformité aux normes légales et éthiques en matière de recherche et d'expérimentation.