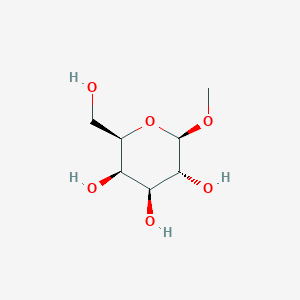
Méthyl β-D-galactopyranoside
Vue d'ensemble
Description
Le β-D-galactopyrannose méthylique est un composé glycoside où un groupe méthyle est lié au carbone anomérique du galactose par une liaison O-glycosidique . C'est un dérivé du galactose, un type de sucre, et il est souvent utilisé dans la recherche biochimique et les applications industrielles.
Applications De Recherche Scientifique
Methyl beta-galactoside has a wide range of applications in scientific research:
Chemistry: Used as a model compound to study glycosidic bond formation and cleavage.
Biology: Employed in studies of carbohydrate metabolism and enzyme activity, particularly beta-galactosidase.
Medicine: Investigated for its potential role in drug delivery systems and as a substrate for diagnostic assays.
Industry: Utilized in the production of prebiotics and as a stabilizer in various formulations
Mécanisme D'action
Target of Action
Methyl β-D-galactopyranoside (MGP) is a carbohydrate ester that has been studied for its potential as an inhibitor for the SARS-CoV-2 protease enzyme . The primary targets of MGP are the prime residues of the SARS-CoV-2 main protease, including Cys145, His41, MET165, GLY143, THR26, and ASN142 . These residues play a crucial role in the protease’s function, which is essential for the life cycle of the virus .
Mode of Action
MGP interacts with its targets through multiple electrostatic and hydrogen bonds . The shape of MGP esters and their ability to form these bonds with the active site of the protease match the binding modes of other minor-groove binders . This interaction inhibits the function of the protease, thereby potentially preventing the replication of the virus .
Biochemical Pathways
MGP is involved in the metabolism of 2-deoxygalactose . When MGP is acylated, it produces 6-O-acyl MGP esters, which are further modified into 2,3,4-tri-O-acyl MGP esters . These esters have a wide variety of functionalities and can affect various biochemical pathways .
Pharmacokinetics
The pharmacokinetic properties of MGP esters have been studied using in silico ADMET (absorption, distribution, metabolism, excretion, and toxicity) prediction . These studies suggest that MGP esters have promising kinetic properties and appear to be safer inhibitors . .
Result of Action
The result of MGP’s action is primarily seen in its antimicrobial effects. In vitro testing against various bacteria and fungi has shown that MGP esters have promising antifungal functionality . Certain compounds were found to be potent against Bacillus subtilis and Escherichia coli strains .
Action Environment
The action of MGP can be influenced by various environmental factors. For instance, the stability of MGP esters can be affected by temperature and pH . Furthermore, the efficacy of MGP as an inhibitor can be influenced by the presence of other molecules in the environment . More research is needed to fully understand how these and other environmental factors influence the action, efficacy, and stability of MGP.
Analyse Biochimique
Biochemical Properties
Methyl beta-D-galactopyranoside is involved in the metabolism of 2-deoxygalactose . It is a hexose, a type of monosaccharide with six carbon atoms, and plays a significant role in the biochemical reactions of certain organisms .
Cellular Effects
The effects of Methyl beta-D-galactopyranoside on various types of cells and cellular processes are complex and multifaceted. It influences cell function, including impacts on cell signaling pathways, gene expression, and cellular metabolism . The specific cellular effects of Methyl beta-D-galactopyranoside can vary widely depending on the type of cell and the specific cellular context .
Molecular Mechanism
The molecular mechanism of action of Methyl beta-D-galactopyranoside involves its interactions with various biomolecules within the cell. It can bind to certain enzymes and proteins, potentially inhibiting or activating them, and can also induce changes in gene expression . The exact nature of these interactions and their consequences can vary depending on the specific molecular context .
Temporal Effects in Laboratory Settings
The effects of Methyl beta-D-galactopyranoside can change over time in laboratory settings. This includes information on the compound’s stability, degradation, and any long-term effects on cellular function observed in in vitro or in vivo studies . The specific temporal effects of Methyl beta-D-galactopyranoside can vary depending on the specific experimental conditions .
Dosage Effects in Animal Models
The effects of Methyl beta-D-galactopyranoside can vary with different dosages in animal models. This could include any threshold effects observed in these studies, as well as any toxic or adverse effects at high doses . The specific dosage effects of Methyl beta-D-galactopyranoside can vary depending on the specific animal model and experimental conditions .
Metabolic Pathways
Methyl beta-D-galactopyranoside is involved in the metabolism of 2-deoxygalactose . It interacts with various enzymes and cofactors within this metabolic pathway . The compound can also have effects on metabolic flux or metabolite levels .
Transport and Distribution
The transport and distribution of Methyl beta-D-galactopyranoside within cells and tissues can vary depending on the specific cellular context . This could include any transporters or binding proteins that it interacts with, as well as any effects on its localization or accumulation .
Subcellular Localization
The subcellular localization of Methyl beta-D-galactopyranoside and any effects on its activity or function can vary depending on the specific cellular context . This could include any targeting signals or post-translational modifications that direct it to specific compartments or organelles .
Méthodes De Préparation
Voies de synthèse et conditions de réaction
Le β-D-galactopyrannose méthylique peut être synthétisé par la réaction du galactose avec du méthanol en présence d'un catalyseur acide . La réaction implique généralement le chauffage du mélange pour faciliter la formation de la liaison glycosidique. Le processus peut être résumé comme suit :
- Dissoudre le galactose dans le méthanol.
- Ajouter un catalyseur acide tel que l'acide chlorhydrique.
- Chauffer le mélange à environ 60 à 70 °C.
- Une fois la réaction terminée, neutraliser l'acide et purifier le produit par cristallisation ou chromatographie.
Méthodes de production industrielles
Dans les milieux industriels, la production de β-D-galactopyrannose méthylique peut impliquer l'utilisation de catalyseurs plus efficaces et de conditions de réaction optimisées pour augmenter le rendement et réduire les coûts . Des méthodes enzymatiques utilisant la β-galactosidase peuvent également être employées pour produire du β-D-galactopyrannose méthylique à partir du lactose ou d'autres galactosides .
Analyse Des Réactions Chimiques
Types de réactions
Le β-D-galactopyrannose méthylique subit diverses réactions chimiques, notamment :
Oxydation : Il peut être oxydé pour former les acides correspondants.
Réduction : Il peut être réduit pour former les alcools correspondants.
Substitution : La liaison glycosidique peut être clivée et substituée par d'autres groupes.
Réactifs et conditions courants
Oxydation : Les oxydants courants comprennent le permanganate de potassium et l'acide nitrique.
Réduction : Des agents réducteurs tels que le borohydrure de sodium peuvent être utilisés.
Substitution : Des conditions acides ou basiques peuvent faciliter le clivage et la substitution de la liaison glycosidique.
Principaux produits formés
Oxydation : Acide galacturonique.
Réduction : Galactitol.
Substitution : Divers galactosides substitués en fonction du substituant utilisé.
Applications de la recherche scientifique
Le β-D-galactopyrannose méthylique a une large gamme d'applications dans la recherche scientifique :
Chimie : Utilisé comme composé modèle pour étudier la formation et le clivage de la liaison glycosidique.
Biologie : Employé dans des études sur le métabolisme des glucides et l'activité enzymatique, en particulier la β-galactosidase.
Médecine : Investigé pour son rôle potentiel dans les systèmes d'administration de médicaments et comme substrat pour les tests diagnostiques.
Industrie : Utilisé dans la production de prébiotiques et comme stabilisateur dans diverses formulations
Mécanisme d'action
Le β-D-galactopyrannose méthylique exerce ses effets principalement par son interaction avec la β-galactosidase, une enzyme qui hydrolyse la liaison glycosidique pour libérer le galactose . Le site actif de l'enzyme se lie à la liaison glycosidique, facilitant son clivage par un mécanisme de double déplacement. Ce processus implique la formation d'un intermédiaire covalent et le transfert du résidu galactose vers une molécule d'eau ou un autre accepteur .
Comparaison Avec Des Composés Similaires
Composés similaires
α-D-galactopyrannose méthylique : Structure similaire mais avec une liaison α-glycosidique.
β-D-galactopyrannose phénylique : Structure similaire mais avec un groupe phényle au lieu d'un groupe méthyle.
β-D-glucopyrannose méthylique : Structure similaire mais avec du glucose au lieu du galactose.
Unicité
Le β-D-galactopyrannose méthylique est unique en raison de son interaction spécifique avec la β-galactosidase et de son rôle dans l'étude du métabolisme des glucides. Son groupe méthyle lui confère une stabilité et en fait un composé modèle utile pour diverses études biochimiques .
Activité Biologique
Methyl beta-D-galactopyranoside (MGP) is a carbohydrate derivative that has garnered attention in medicinal chemistry due to its potential biological activities, particularly in antimicrobial and anticancer applications. This article reviews the biological activity of MGP, synthesizing findings from various studies to provide a comprehensive overview.
MGP is a methyl ester of beta-D-galactopyranoside, which can be synthesized through various methods, including acylation reactions. Recent studies have focused on modifying MGP to enhance its biological efficacy. For instance, MGP esters were synthesized using acyl halides, resulting in compounds with improved antimicrobial properties against various pathogens .
Antimicrobial Activity
MGP and its derivatives have shown significant antimicrobial activity. A study evaluating the antimicrobial effects of synthesized MGP esters reported promising results against both Gram-positive and Gram-negative bacteria, as well as fungi. The minimum inhibitory concentration (MIC) values for the most potent compounds ranged from 0.352 ± 0.02 to 0.703 ± 0.01 mg/ml, while minimum bactericidal concentration (MBC) values were between 0.704 ± 0.02 and 1.408 ± 0.04 mg/ml .
Table 1: Antimicrobial Activity of MGP Derivatives
Compound | MIC (mg/ml) | MBC (mg/ml) | Target Pathogen |
---|---|---|---|
Compound 3 | 0.352 ± 0.02 | 0.704 ± 0.02 | Bacillus subtilis |
Compound 10 | 0.703 ± 0.01 | 1.408 ± 0.04 | Escherichia coli |
Other Esters | Varies | Varies | Various Gram-positive and Gram-negative bacteria |
The mechanism by which MGP exhibits its antimicrobial activity involves the disruption of microbial cell membranes and interference with metabolic pathways. Molecular docking studies have indicated that MGP esters interact effectively with the active sites of microbial proteins, enhancing their binding affinity and stability . The computational analysis using density functional theory (DFT) further supports these findings by demonstrating the thermodynamic stability of these compounds compared to the parent molecule.
Anticancer Properties
In addition to its antimicrobial properties, MGP has been investigated for its anticancer potential. Research indicates that certain derivatives of MGP exhibit cytotoxic effects against various cancer cell lines, attributed to their ability to induce apoptosis and inhibit cell proliferation . The structure-activity relationship (SAR) studies suggest that modifications to the acyl chain can significantly enhance the anticancer activity of MGP derivatives.
Case Studies
- SARS-CoV-2 Inhibition : Recent studies have explored the potential of MGP derivatives as inhibitors of the SARS-CoV-2 main protease, crucial for viral replication. Molecular docking simulations revealed that several modified MGP esters bind effectively to key residues in the protease's active site, suggesting their potential as therapeutic agents against COVID-19 .
- Antioxidant Activity : In vitro assays have demonstrated that MGP possesses antioxidant properties, contributing to its protective effects against oxidative stress in biological systems . This activity is likely linked to its structural characteristics, which allow it to scavenge free radicals effectively.
Pharmacokinetics and Safety
The pharmacokinetic profiles of MGP derivatives have been assessed using in silico models, indicating favorable absorption, distribution, metabolism, excretion, and toxicity (ADMET) properties . These findings suggest that modified MGP compounds could be safer alternatives for therapeutic applications.
Propriétés
IUPAC Name |
(2R,3R,4S,5R,6R)-2-(hydroxymethyl)-6-methoxyoxane-3,4,5-triol | |
---|---|---|
Source | PubChem | |
URL | https://pubchem.ncbi.nlm.nih.gov | |
Description | Data deposited in or computed by PubChem | |
InChI |
InChI=1S/C7H14O6/c1-12-7-6(11)5(10)4(9)3(2-8)13-7/h3-11H,2H2,1H3/t3-,4+,5+,6-,7-/m1/s1 | |
Source | PubChem | |
URL | https://pubchem.ncbi.nlm.nih.gov | |
Description | Data deposited in or computed by PubChem | |
InChI Key |
HOVAGTYPODGVJG-VOQCIKJUSA-N | |
Source | PubChem | |
URL | https://pubchem.ncbi.nlm.nih.gov | |
Description | Data deposited in or computed by PubChem | |
Canonical SMILES |
COC1C(C(C(C(O1)CO)O)O)O | |
Source | PubChem | |
URL | https://pubchem.ncbi.nlm.nih.gov | |
Description | Data deposited in or computed by PubChem | |
Isomeric SMILES |
CO[C@H]1[C@@H]([C@H]([C@H]([C@H](O1)CO)O)O)O | |
Source | PubChem | |
URL | https://pubchem.ncbi.nlm.nih.gov | |
Description | Data deposited in or computed by PubChem | |
Molecular Formula |
C7H14O6 | |
Source | PubChem | |
URL | https://pubchem.ncbi.nlm.nih.gov | |
Description | Data deposited in or computed by PubChem | |
DSSTOX Substance ID |
DTXSID901017001 | |
Record name | Methyl galactoside | |
Source | EPA DSSTox | |
URL | https://comptox.epa.gov/dashboard/DTXSID901017001 | |
Description | DSSTox provides a high quality public chemistry resource for supporting improved predictive toxicology. | |
Molecular Weight |
194.18 g/mol | |
Source | PubChem | |
URL | https://pubchem.ncbi.nlm.nih.gov | |
Description | Data deposited in or computed by PubChem | |
CAS No. |
1824-94-8 | |
Record name | Methyl β-D-galactopyranoside | |
Source | CAS Common Chemistry | |
URL | https://commonchemistry.cas.org/detail?cas_rn=1824-94-8 | |
Description | CAS Common Chemistry is an open community resource for accessing chemical information. Nearly 500,000 chemical substances from CAS REGISTRY cover areas of community interest, including common and frequently regulated chemicals, and those relevant to high school and undergraduate chemistry classes. This chemical information, curated by our expert scientists, is provided in alignment with our mission as a division of the American Chemical Society. | |
Explanation | The data from CAS Common Chemistry is provided under a CC-BY-NC 4.0 license, unless otherwise stated. | |
Record name | Methyl beta-galactoside | |
Source | ChemIDplus | |
URL | https://pubchem.ncbi.nlm.nih.gov/substance/?source=chemidplus&sourceid=0001824948 | |
Description | ChemIDplus is a free, web search system that provides access to the structure and nomenclature authority files used for the identification of chemical substances cited in National Library of Medicine (NLM) databases, including the TOXNET system. | |
Record name | Methyl galactoside | |
Source | EPA DSSTox | |
URL | https://comptox.epa.gov/dashboard/DTXSID901017001 | |
Description | DSSTox provides a high quality public chemistry resource for supporting improved predictive toxicology. | |
Record name | Methyl β-D-galactopyranoside | |
Source | European Chemicals Agency (ECHA) | |
URL | https://echa.europa.eu/substance-information/-/substanceinfo/100.015.783 | |
Description | The European Chemicals Agency (ECHA) is an agency of the European Union which is the driving force among regulatory authorities in implementing the EU's groundbreaking chemicals legislation for the benefit of human health and the environment as well as for innovation and competitiveness. | |
Explanation | Use of the information, documents and data from the ECHA website is subject to the terms and conditions of this Legal Notice, and subject to other binding limitations provided for under applicable law, the information, documents and data made available on the ECHA website may be reproduced, distributed and/or used, totally or in part, for non-commercial purposes provided that ECHA is acknowledged as the source: "Source: European Chemicals Agency, http://echa.europa.eu/". Such acknowledgement must be included in each copy of the material. ECHA permits and encourages organisations and individuals to create links to the ECHA website under the following cumulative conditions: Links can only be made to webpages that provide a link to the Legal Notice page. | |
Record name | METHYL .BETA.-D-GALACTOPYRANOSIDE | |
Source | FDA Global Substance Registration System (GSRS) | |
URL | https://gsrs.ncats.nih.gov/ginas/app/beta/substances/64RYD088RJ | |
Description | The FDA Global Substance Registration System (GSRS) enables the efficient and accurate exchange of information on what substances are in regulated products. Instead of relying on names, which vary across regulatory domains, countries, and regions, the GSRS knowledge base makes it possible for substances to be defined by standardized, scientific descriptions. | |
Explanation | Unless otherwise noted, the contents of the FDA website (www.fda.gov), both text and graphics, are not copyrighted. They are in the public domain and may be republished, reprinted and otherwise used freely by anyone without the need to obtain permission from FDA. Credit to the U.S. Food and Drug Administration as the source is appreciated but not required. | |
Retrosynthesis Analysis
AI-Powered Synthesis Planning: Our tool employs the Template_relevance Pistachio, Template_relevance Bkms_metabolic, Template_relevance Pistachio_ringbreaker, Template_relevance Reaxys, Template_relevance Reaxys_biocatalysis model, leveraging a vast database of chemical reactions to predict feasible synthetic routes.
One-Step Synthesis Focus: Specifically designed for one-step synthesis, it provides concise and direct routes for your target compounds, streamlining the synthesis process.
Accurate Predictions: Utilizing the extensive PISTACHIO, BKMS_METABOLIC, PISTACHIO_RINGBREAKER, REAXYS, REAXYS_BIOCATALYSIS database, our tool offers high-accuracy predictions, reflecting the latest in chemical research and data.
Strategy Settings
Precursor scoring | Relevance Heuristic |
---|---|
Min. plausibility | 0.01 |
Model | Template_relevance |
Template Set | Pistachio/Bkms_metabolic/Pistachio_ringbreaker/Reaxys/Reaxys_biocatalysis |
Top-N result to add to graph | 6 |
Feasible Synthetic Routes
Q1: How does methyl beta-D-galactopyranoside interact with lectins?
A1: Methyl beta-D-galactopyranoside binds to lectins, a diverse group of carbohydrate-binding proteins, through specific interactions with its galactose moiety. The strength of binding varies depending on the specific lectin and the orientation of the methyl group (alpha or beta). For example, it demonstrates higher affinity for the lectin Ricinus communis agglutinin I (RCA120) in the beta anomeric configuration. [] This interaction primarily involves the hydroxyl groups at the C2, C3, and C4 positions of the galactose ring. [, ]
Q2: What are the structural features of methyl beta-D-galactopyranoside?
A2:
- Spectroscopic Data: Detailed 1H and 13C NMR data for methyl beta-D-galactopyranoside and its derivatives can be found in several research articles. [, , ] These studies provide valuable information about the compound's conformation and structural features.
Q3: Does the conformation of methyl beta-D-galactopyranoside influence its binding to proteins?
A3: Yes, the conformation of the glycosidic linkage in methyl beta-D-galactopyranoside can influence its binding affinity to proteins like lectins. This is evident from the different binding affinities observed for its alpha and beta anomers with certain lectins. [, , ]
Q4: Have there been any computational studies investigating the interactions of methyl beta-D-galactopyranoside with proteins?
A6: Yes, computational studies, including molecular modeling and theoretical calculations, have been employed to understand the interactions between methyl beta-D-galactopyranoside and aromatic compounds, particularly focusing on the CH/pi interaction. [, ] These studies provide insights into the nature and strength of these interactions, which are relevant for understanding carbohydrate-protein interactions in biological systems.
Q5: How do modifications to the galactose moiety of methyl beta-D-galactopyranoside affect its biological activity?
A7: Modifications to the galactose moiety can significantly influence the binding affinity and selectivity of methyl beta-D-galactopyranoside towards different proteins. For instance, the introduction of hydrophobic substituents, such as cyclohexyl or phenyl groups, at the alpha position of the galactose ring can enhance binding affinity to the lactose permease of Escherichia coli. [] Additionally, modifications at the C6 position, such as deoxygenation or halogenation, have been shown to impact the transport of galactose analogs in biological systems. []
Q6: Are there specific structural requirements for the recognition of methyl beta-D-galactopyranoside by the lactose permease of Escherichia coli?
A8: Yes, the lactose permease of E. coli exhibits a distinct preference for alpha-substituted D-galactopyranosides with hydrophobic aglycons. The presence of bulky hydrophobic groups at the alpha position enhances binding affinity, suggesting that the interaction is directed towards the carbon atom linked to the anomeric oxygen. []
Q7: What analytical techniques are commonly used to study methyl beta-D-galactopyranoside?
A7: Various analytical techniques are employed to characterize and study methyl beta-D-galactopyranoside:
- Nuclear Magnetic Resonance (NMR) Spectroscopy: Provides detailed structural information and is frequently used to study the compound's conformation and interactions with other molecules. [, , ]
- High-Performance Liquid Chromatography (HPLC): Allows for the separation and quantification of methyl beta-D-galactopyranoside and its derivatives. []
- Gas Chromatography-Mass Spectrometry (GC-MS): Enables the identification and quantification of methyl beta-D-galactopyranoside in complex mixtures. []
- Enzyme Assays: Utilized to investigate the role of methyl beta-D-galactopyranoside as a substrate or inhibitor for enzymes such as beta-galactosidases. [, ]
- Haemagglutination (HA) and HA Inhibition Assays: Employed to study the interactions of methyl beta-D-galactopyranoside with lectins and assess their binding affinity. []
Q8: What is the role of methyl beta-D-galactopyranoside in bacterial adhesion?
A10: Research suggests that some bacteria, like Vibrio shiloi, adhere to their host using a beta-D-galactopyranoside-containing receptor. [] This adhesion can be inhibited by the presence of methyl-beta-D-galactopyranoside, indicating its involvement in the recognition process.
Q9: How is the synthesis of methyl 6-O-alpha-D-galactopyranosyl-beta-D-galactopyranoside achieved?
A11: This disaccharide can be synthesized through a multi-step process involving the reaction of methyl 2,3,4-tri-O-acetyl-beta-D-galactopyranoside with 2,3,4,6-tetra-O-acetyl-alpha-D-galactosyl bromide, followed by deacetylation. []
Avertissement et informations sur les produits de recherche in vitro
Veuillez noter que tous les articles et informations sur les produits présentés sur BenchChem sont destinés uniquement à des fins informatives. Les produits disponibles à l'achat sur BenchChem sont spécifiquement conçus pour des études in vitro, qui sont réalisées en dehors des organismes vivants. Les études in vitro, dérivées du terme latin "in verre", impliquent des expériences réalisées dans des environnements de laboratoire contrôlés à l'aide de cellules ou de tissus. Il est important de noter que ces produits ne sont pas classés comme médicaments et n'ont pas reçu l'approbation de la FDA pour la prévention, le traitement ou la guérison de toute condition médicale, affection ou maladie. Nous devons souligner que toute forme d'introduction corporelle de ces produits chez les humains ou les animaux est strictement interdite par la loi. Il est essentiel de respecter ces directives pour assurer la conformité aux normes légales et éthiques en matière de recherche et d'expérimentation.