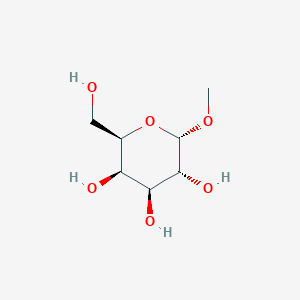
Méthyl α-D-galactopyranoside
Vue d'ensemble
Description
Methyl alpha-D-galactoside is an alpha-D-galactoside having a methyl substituent at the anomeric position. It is an alpha-D-galactoside, a methyl D-galactoside and a monosaccharide derivative.
Methyl alpha-D-galactopyranoside is a natural product found in Ligustrum obtusifolium, Perilla frutescens, and Cichorium intybus with data available.
Applications De Recherche Scientifique
Agents antimicrobiens
Les esters de MDG ont montré des résultats prometteurs en tant qu'agents antimicrobiens. Ils se sont avérés avoir une meilleure activité antifongique que les agents antibactériens . En particulier, l'ester MDG 7 – 12 a montré une énergie de liaison plus élevée que le médicament antifongique, le fluconazole .
Médicaments antiviraux
Les esters de MDG se sont également avérés avoir une énergie de liaison prometteuse avec la principale protéase du SRAS-CoV-2 (6LU7) que la tétracycline, le fluconazole et l'inhibiteur natif N3 . Cela suggère que les esters de MDG pourraient potentiellement être utilisés comme médicaments antiviraux.
Évaluation de l'aspect médicament
La plupart des esters de MDG satisfont l'évaluation de l'aspect médicament, les tests de biodisponibilité et de sécurité . Cela indique que ces composés pourraient potentiellement être développés en médicaments.
Inhibiteur des α-galactosidases
Le MDG est un puissant inhibiteur contre les α-galactosidases extracellulaires et intracellulaires de Debaryomyces hansenii UFV-1 . Cela pourrait être utile pour contrôler l'activité de ces enzymes.
Études computationnelles
Le MDG a été utilisé dans des études computationnelles du β-d-galactose protoné et de son complexe hydraté . Cela contribue à comprendre les propriétés et le comportement de ces molécules.
Synthèse de α-D-galactopyranosides de méthyle monosulfatés
Le MDG a été utilisé dans la synthèse de α-D-galactopyranosides de méthyle monosulfatés . Ces composés ont une large distribution dans les plantes et les animaux et sont impliqués dans divers processus biologiques .
Mécanisme D'action
Target of Action
Methyl alpha-D-galactopyranoside primarily targets the extracellular and intracellular α-galactosidases of the yeast Debaryomyces hansenii UFV-1 . These enzymes are responsible for the hydrolysis of alpha-galactosides, a type of sugar molecule, into monosaccharides.
Mode of Action
Methyl alpha-D-galactopyranoside acts as a potent inhibitor against these α-galactosidases . It binds to the active sites of these enzymes, preventing them from catalyzing the breakdown of alpha-galactosides. This interaction alters the normal function of the enzymes, leading to changes in the metabolic processes within the yeast cells.
Pharmacokinetics
It is known to be soluble in water , which could influence its bioavailability and distribution within biological systems.
Result of Action
The primary result of Methyl alpha-D-galactopyranoside’s action is the inhibition of α-galactosidases in Debaryomyces hansenii UFV-1, leading to changes in the yeast’s metabolic processes . The specific molecular and cellular effects depend on the role of these enzymes in the yeast’s metabolism and require further investigation.
Analyse Biochimique
Biochemical Properties
Methyl alpha-D-galactopyranoside plays a significant role in biochemical reactions, particularly as an inhibitor of certain enzymes. It is known to inhibit the activity of Debaryomyces hansenii UFV-1 extracellular and intracellular alpha-galactosidases . These enzymes are responsible for breaking down complex carbohydrates into simpler sugars. By inhibiting these enzymes, Methyl alpha-D-galactopyranoside can be used to study the metabolic pathways involving galactose and its derivatives.
Cellular Effects
Methyl alpha-D-galactopyranoside has various effects on different types of cells and cellular processes. It influences cell function by interfering with carbohydrate metabolism, which can impact cell signaling pathways, gene expression, and cellular metabolism. For instance, its inhibitory effect on alpha-galactosidases can lead to an accumulation of galactose-containing compounds within cells, potentially altering cellular activities and metabolic fluxes .
Molecular Mechanism
At the molecular level, Methyl alpha-D-galactopyranoside exerts its effects primarily through enzyme inhibition. It binds to the active sites of alpha-galactosidases, preventing these enzymes from catalyzing the hydrolysis of galactose-containing substrates . This binding interaction is crucial for understanding how this compound can modulate biochemical pathways and cellular functions.
Temporal Effects in Laboratory Settings
In laboratory settings, the effects of Methyl alpha-D-galactopyranoside can change over time. The compound’s stability and degradation are important factors to consider. Studies have shown that it remains stable under standard storage conditions, but its long-term effects on cellular function can vary depending on the experimental setup. In vitro and in vivo studies have demonstrated that prolonged exposure to Methyl alpha-D-galactopyranoside can lead to sustained inhibition of alpha-galactosidases, affecting cellular metabolism over time .
Dosage Effects in Animal Models
The effects of Methyl alpha-D-galactopyranoside in animal models vary with different dosages. At lower doses, it can effectively inhibit alpha-galactosidases without causing significant adverse effects. At higher doses, there may be toxic or adverse effects, including disruptions in carbohydrate metabolism and potential toxicity to certain tissues . These dosage-dependent effects are crucial for determining the safe and effective use of this compound in research.
Metabolic Pathways
Methyl alpha-D-galactopyranoside is involved in metabolic pathways related to carbohydrate metabolism. It interacts with enzymes such as alpha-galactosidases, which play a key role in the breakdown of galactose-containing compounds . By inhibiting these enzymes, Methyl alpha-D-galactopyranoside can alter metabolic fluxes and affect the levels of various metabolites within cells.
Transport and Distribution
Within cells and tissues, Methyl alpha-D-galactopyranoside is transported and distributed through specific transporters and binding proteins. These interactions can influence its localization and accumulation in different cellular compartments. Understanding the transport mechanisms of this compound is essential for elucidating its effects on cellular functions and metabolic pathways .
Subcellular Localization
The subcellular localization of Methyl alpha-D-galactopyranoside is determined by targeting signals and post-translational modifications. These factors direct the compound to specific compartments or organelles within the cell, where it can exert its inhibitory effects on alpha-galactosidases . The precise localization of this compound is important for understanding its role in cellular metabolism and enzyme regulation.
Propriétés
IUPAC Name |
(2R,3R,4S,5R,6S)-2-(hydroxymethyl)-6-methoxyoxane-3,4,5-triol | |
---|---|---|
Source | PubChem | |
URL | https://pubchem.ncbi.nlm.nih.gov | |
Description | Data deposited in or computed by PubChem | |
InChI |
InChI=1S/C7H14O6/c1-12-7-6(11)5(10)4(9)3(2-8)13-7/h3-11H,2H2,1H3/t3-,4+,5+,6-,7+/m1/s1 | |
Source | PubChem | |
URL | https://pubchem.ncbi.nlm.nih.gov | |
Description | Data deposited in or computed by PubChem | |
InChI Key |
HOVAGTYPODGVJG-PZRMXXKTSA-N | |
Source | PubChem | |
URL | https://pubchem.ncbi.nlm.nih.gov | |
Description | Data deposited in or computed by PubChem | |
Canonical SMILES |
COC1C(C(C(C(O1)CO)O)O)O | |
Source | PubChem | |
URL | https://pubchem.ncbi.nlm.nih.gov | |
Description | Data deposited in or computed by PubChem | |
Isomeric SMILES |
CO[C@@H]1[C@@H]([C@H]([C@H]([C@H](O1)CO)O)O)O | |
Source | PubChem | |
URL | https://pubchem.ncbi.nlm.nih.gov | |
Description | Data deposited in or computed by PubChem | |
Molecular Formula |
C7H14O6 | |
Source | PubChem | |
URL | https://pubchem.ncbi.nlm.nih.gov | |
Description | Data deposited in or computed by PubChem | |
DSSTOX Substance ID |
DTXSID30187575 | |
Record name | Methyl alpha-galactopyranoside | |
Source | EPA DSSTox | |
URL | https://comptox.epa.gov/dashboard/DTXSID30187575 | |
Description | DSSTox provides a high quality public chemistry resource for supporting improved predictive toxicology. | |
Molecular Weight |
194.18 g/mol | |
Source | PubChem | |
URL | https://pubchem.ncbi.nlm.nih.gov | |
Description | Data deposited in or computed by PubChem | |
CAS No. |
3396-99-4, 34004-14-3 | |
Record name | Methyl α-D-galactopyranoside | |
Source | CAS Common Chemistry | |
URL | https://commonchemistry.cas.org/detail?cas_rn=3396-99-4 | |
Description | CAS Common Chemistry is an open community resource for accessing chemical information. Nearly 500,000 chemical substances from CAS REGISTRY cover areas of community interest, including common and frequently regulated chemicals, and those relevant to high school and undergraduate chemistry classes. This chemical information, curated by our expert scientists, is provided in alignment with our mission as a division of the American Chemical Society. | |
Explanation | The data from CAS Common Chemistry is provided under a CC-BY-NC 4.0 license, unless otherwise stated. | |
Record name | Methyl-galactopyranoside | |
Source | ChemIDplus | |
URL | https://pubchem.ncbi.nlm.nih.gov/substance/?source=chemidplus&sourceid=0003396994 | |
Description | ChemIDplus is a free, web search system that provides access to the structure and nomenclature authority files used for the identification of chemical substances cited in National Library of Medicine (NLM) databases, including the TOXNET system. | |
Record name | Methyl alpha-galactoside | |
Source | DrugBank | |
URL | https://www.drugbank.ca/drugs/DB02100 | |
Description | The DrugBank database is a unique bioinformatics and cheminformatics resource that combines detailed drug (i.e. chemical, pharmacological and pharmaceutical) data with comprehensive drug target (i.e. sequence, structure, and pathway) information. | |
Explanation | Creative Common's Attribution-NonCommercial 4.0 International License (http://creativecommons.org/licenses/by-nc/4.0/legalcode) | |
Record name | Methyl alpha-galactopyranoside | |
Source | EPA DSSTox | |
URL | https://comptox.epa.gov/dashboard/DTXSID30187575 | |
Description | DSSTox provides a high quality public chemistry resource for supporting improved predictive toxicology. | |
Record name | Methyl α-D-galactopyranoside | |
Source | European Chemicals Agency (ECHA) | |
URL | https://echa.europa.eu/substance-information/-/substanceinfo/100.020.229 | |
Description | The European Chemicals Agency (ECHA) is an agency of the European Union which is the driving force among regulatory authorities in implementing the EU's groundbreaking chemicals legislation for the benefit of human health and the environment as well as for innovation and competitiveness. | |
Explanation | Use of the information, documents and data from the ECHA website is subject to the terms and conditions of this Legal Notice, and subject to other binding limitations provided for under applicable law, the information, documents and data made available on the ECHA website may be reproduced, distributed and/or used, totally or in part, for non-commercial purposes provided that ECHA is acknowledged as the source: "Source: European Chemicals Agency, http://echa.europa.eu/". Such acknowledgement must be included in each copy of the material. ECHA permits and encourages organisations and individuals to create links to the ECHA website under the following cumulative conditions: Links can only be made to webpages that provide a link to the Legal Notice page. | |
Record name | Methyl-α-D-galactose | |
Source | FDA Global Substance Registration System (GSRS) | |
URL | https://gsrs.ncats.nih.gov/ginas/app/beta/substances/ESJ6UY55QN | |
Description | The FDA Global Substance Registration System (GSRS) enables the efficient and accurate exchange of information on what substances are in regulated products. Instead of relying on names, which vary across regulatory domains, countries, and regions, the GSRS knowledge base makes it possible for substances to be defined by standardized, scientific descriptions. | |
Explanation | Unless otherwise noted, the contents of the FDA website (www.fda.gov), both text and graphics, are not copyrighted. They are in the public domain and may be republished, reprinted and otherwise used freely by anyone without the need to obtain permission from FDA. Credit to the U.S. Food and Drug Administration as the source is appreciated but not required. | |
Retrosynthesis Analysis
AI-Powered Synthesis Planning: Our tool employs the Template_relevance Pistachio, Template_relevance Bkms_metabolic, Template_relevance Pistachio_ringbreaker, Template_relevance Reaxys, Template_relevance Reaxys_biocatalysis model, leveraging a vast database of chemical reactions to predict feasible synthetic routes.
One-Step Synthesis Focus: Specifically designed for one-step synthesis, it provides concise and direct routes for your target compounds, streamlining the synthesis process.
Accurate Predictions: Utilizing the extensive PISTACHIO, BKMS_METABOLIC, PISTACHIO_RINGBREAKER, REAXYS, REAXYS_BIOCATALYSIS database, our tool offers high-accuracy predictions, reflecting the latest in chemical research and data.
Strategy Settings
Precursor scoring | Relevance Heuristic |
---|---|
Min. plausibility | 0.01 |
Model | Template_relevance |
Template Set | Pistachio/Bkms_metabolic/Pistachio_ringbreaker/Reaxys/Reaxys_biocatalysis |
Top-N result to add to graph | 6 |
Feasible Synthetic Routes
Avertissement et informations sur les produits de recherche in vitro
Veuillez noter que tous les articles et informations sur les produits présentés sur BenchChem sont destinés uniquement à des fins informatives. Les produits disponibles à l'achat sur BenchChem sont spécifiquement conçus pour des études in vitro, qui sont réalisées en dehors des organismes vivants. Les études in vitro, dérivées du terme latin "in verre", impliquent des expériences réalisées dans des environnements de laboratoire contrôlés à l'aide de cellules ou de tissus. Il est important de noter que ces produits ne sont pas classés comme médicaments et n'ont pas reçu l'approbation de la FDA pour la prévention, le traitement ou la guérison de toute condition médicale, affection ou maladie. Nous devons souligner que toute forme d'introduction corporelle de ces produits chez les humains ou les animaux est strictement interdite par la loi. Il est essentiel de respecter ces directives pour assurer la conformité aux normes légales et éthiques en matière de recherche et d'expérimentation.