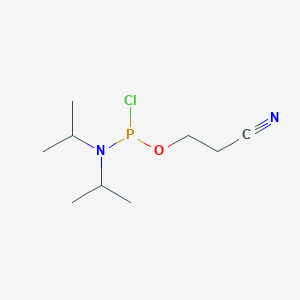
2-Cyanoethyl N,N-diisopropylchlorophosphoramidite
Vue d'ensemble
Description
2-Cyanoethyl N,N-diisopropylchlorophosphoramidite (CAS 89992-70-1) is a key reagent in oligonucleotide synthesis, particularly for introducing phosphoramidite linkages during solid-phase DNA/RNA assembly. Its molecular formula is C₉H₁₈ClN₂OP (MW 236.68), with a density of 1.061 g/mL at 25°C and a boiling point of 103–105°C . The compound is a moisture-sensitive liquid, requiring storage at 2–8°C or –20°C to prevent decomposition . It reacts violently with water, releasing toxic gases (e.g., HCl and HCN), and is incompatible with alcohols, strong bases, and oxidizers .
Its primary application lies in synthesizing modified nucleosides, such as Locked Nucleic Acids (LNAs) and fluorinated nucleotides, where it facilitates phosphitylation of hydroxyl groups . Safety protocols mandate handling in chemical fume hoods with personal protective equipment (PPE) due to its corrosive (Skin Corrosion 1B) and flammable nature (flash point: 110°C) .
Méthodes De Préparation
Traditional Laboratory Synthesis
Reagents and Reaction Conditions
The conventional synthesis involves reacting chlorophosphite derivatives with 2-cyanoethyl diisopropylamine under anhydrous conditions. Key reagents include:
-
Chlorophosphite precursors : Typically derived from phosphorus trichloride (PCl₃) and alcohols .
-
2-Cyanoethyl diisopropylamine : Serves as the nucleophile for phosphitylation .
-
Diisopropylethylamine (DIPEA) : Used as a base to neutralize HCl byproducts .
Reactions are conducted in anhydrous dichloromethane (DCM) under a nitrogen atmosphere to prevent hydrolysis . Temperatures are maintained at 0–25°C to balance reactivity and side-product formation .
Step-by-Step Procedure
-
Chlorophosphite Preparation : PCl₃ is reacted with an alcohol (e.g., 2-cyanoethanol) in DCM at −78°C to form chlorophosphite intermediates .
-
Phosphitylation : Chlorophosphite is added dropwise to a solution of 2-cyanoethyl diisopropylamine and DIPEA in DCM. The mixture is stirred for 1–2 hours at room temperature .
-
Quenching : Excess reagents are neutralized with 5% sodium bicarbonate, followed by washing with water .
Purification Techniques
Crude product is purified via:
-
Flash chromatography : Silica gel columns eluted with 40% ethyl acetate and 1% triethylamine in hexanes .
-
Drying : Anhydrous sodium sulfate is used to remove residual moisture before solvent evaporation .
-
Vacuum distillation : Removes low-boiling impurities, yielding a colorless liquid .
Alternative Synthetic Approaches
Ionic Liquid-Mediated Synthesis
Recent advancements utilize ionic liquids (e.g., [C₄mim][NTf₂]) as reaction media to enhance efficiency :
-
Procedure : Chlorophosphite, 2-cyanoethyl diisopropylamine, and DIPEA are mixed in the ionic liquid at 25°C for 3 hours .
-
Advantages :
Solventless and Mechanochemical Methods
-
Ball milling : Reagents are ground in a high-energy mill for 1.5 hours, achieving 85% yield without solvents .
-
Sonication : Ultrasonic irradiation accelerates reaction kinetics, completing phosphitylation in 30 minutes .
Industrial-Scale Production
Scalable Synthesis Techniques
Industrial protocols emphasize batch reproducibility and safety:
-
Reactor Design : Glass-lined vessels with nitrogen purging and temperature control .
-
Process Optimization :
Yield Optimization and Quality Control
-
Distillation : High-purity (>98%) product is obtained via fractional distillation under reduced pressure (0.8 mmHg, 103–105°C) .
-
Stability Testing : Batches are stored at −20°C under argon to prevent degradation .
Purification Method | Conditions | Purity Achieved | Source |
---|---|---|---|
Flash chromatography | 40% ethyl acetate/hexanes + 1% triethylamine | >95% | |
Vacuum distillation | 0.8 mmHg, 103–105°C | >98% |
Comparative Analysis of Synthesis Methods
Reaction Efficiency
Method | Time (h) | Yield (%) | Purity (%) |
---|---|---|---|
Traditional | 2 | 70–75 | 95 |
Ionic Liquid | 3 | 80–85 | 97 |
Ball Milling | 1.5 | 85 | 96 |
Challenges and Mitigation Strategies
Side Reactions
-
Hydrolysis : Controlled by rigorous drying of reagents and solvents .
-
Oligomerization : Minimized via low-temperature (−78°C) chlorophosphite synthesis .
Scalability Limitations
Analyse Des Réactions Chimiques
Types of Reactions
2-Cyanoethyl N,N-diisopropylchlorophosphoramidite undergoes various chemical reactions, including:
Substitution Reactions: It can participate in nucleophilic substitution reactions where the chlorine atom is replaced by other nucleophiles.
Phosphorylation Reactions: It is commonly used in phosphorylation reactions to introduce phosphate groups into organic molecules.
Common Reagents and Conditions
Nucleophilic Substitution: Common nucleophiles used in substitution reactions include amines and alcohols. The reactions
Activité Biologique
2-Cyanoethyl N,N-diisopropylchlorophosphoramidite is a phosphoramidite compound widely utilized in the synthesis of oligonucleotides, particularly in the context of RNA interference (RNAi) and other nucleic acid-based therapeutics. Its biological activity primarily revolves around its role as a building block for the formation of oligonucleotides that can modulate gene expression. This article reviews the synthesis, applications, and biological evaluation of this compound, highlighting key research findings and case studies.
This compound has the following chemical characteristics:
- Molecular Formula : C₉H₁₈ClN₂O₂P
- Molecular Weight : 236.68 g/mol
- CAS Number : 89992-70-1
This compound is known for its high reactivity, which facilitates the phosphorylation of nucleosides during oligonucleotide synthesis. However, its instability at room temperature and tendency to undergo undesired reactions with nucleobases pose challenges in its application .
The synthesis of this compound typically involves the reaction of diisopropylamine with chlorophosphoryl cyanide. This process results in a highly reactive phosphoramidite that can be utilized in automated solid-phase oligonucleotide synthesis .
The mechanism by which this compound exerts its biological effects is primarily through the formation of phosphodiester bonds in nucleic acids. When incorporated into oligonucleotides, it enables the production of small interfering RNAs (siRNAs) that can effectively silence target genes by promoting RNA degradation via the RNA-induced silencing complex (RISC) .
RNA Interference
One of the most significant applications of this compound is in the field of RNA interference (RNAi). Research has demonstrated that siRNAs synthesized using this phosphoramidite can effectively reduce off-target effects while maintaining on-target activity. For instance, studies have shown that introducing derivatives into siRNA strands can lead to a significant suppression of off-target activity without compromising gene silencing efficacy .
Case Studies
- siRNA Development : In a study focused on developing siRNAs with enhanced specificity, researchers incorporated this compound into various nucleoside sequences. The resulting siRNAs demonstrated improved performance in cellular assays, effectively silencing target genes while minimizing unintended interactions with non-target sequences .
- Photoregulated Gene Silencing : Another innovative application involved synthesizing photo-switchable siRNAs using this phosphoramidite. These siRNAs could be activated or deactivated by exposure to specific wavelengths of light, providing a novel approach to control gene expression temporally .
Efficacy and Stability
Research indicates that while this compound allows for efficient oligonucleotide synthesis, it also presents challenges related to stability and reactivity. Comparative studies have shown that newer phosphoramidites, such as 2-cyanoethyl N,N,N',N'-tetraisopropylphosphoramidite, may offer improved yields and lower reactivity issues during synthesis .
Comparative Table of Phosphoramidites
Property | This compound | 2-Cyanoethyl N,N,N',N'-Tetraisopropylphosphoramidite |
---|---|---|
Stability | Moderate; sensitive to moisture and temperature | Higher; more stable under standard conditions |
Yield | Variable; often lower due to side reactions | Generally higher; fewer undesired byproducts |
Reactivity | High; prone to side reactions with nucleobases | Lower; more controlled reactivity |
Applications De Recherche Scientifique
Key Applications
-
Oligonucleotide Synthesis
- Phosphitylation of Hydroxyl Groups : This compound is employed to selectively phosphorylate the 3′-hydroxyl groups of nucleosides during oligonucleotide synthesis. It facilitates the formation of phosphoramidites, which are crucial intermediates in the solid-phase synthesis of DNA and RNA oligonucleotides .
- LNA Phosphoramidites : Recent studies have demonstrated its use in synthesizing locked nucleic acid (LNA) phosphoramidites, which enhance the stability and binding affinity of oligonucleotides .
- Selective Monophosphorylation
- Conversion of Protected Ribonucleosides
- Scalable Oligonucleotide Synthesis
Case Study 1: Synthesis of LNA Oligonucleotides
In a recent patent study, researchers utilized this compound to synthesize LNA amidites with improved yields and purity compared to previous methods. The study highlighted the efficiency of using this compound in conjunction with nucleophilic activators like 4,5-dicyanoimidazole, resulting in high-quality LNA oligonucleotides suitable for therapeutic applications .
Case Study 2: Monophosphorylation of Carbohydrates
Another application involved the selective monophosphorylation of various carbohydrates using this phosphoramidite. The study demonstrated that the compound could selectively modify hydroxyl groups without affecting other functional groups, showcasing its utility in carbohydrate chemistry and potential applications in drug development .
Data Tables
Hazard Category | Signal Word | Precautionary Measures |
---|---|---|
Serious eye damage | Danger | Wear eye protection |
Skin corrosion/irritation | Danger | Use protective gloves |
Pyrophoric liquid | Danger | Handle under inert gas |
Q & A
Basic Questions
Q. What are the critical handling and storage requirements for 2-cyanoethyl N,N-diisopropylchlorophosphoramidite to ensure stability?
The compound is highly moisture-sensitive and reacts with water to release toxic gases . Store at 2–8°C in airtight containers under inert gas (e.g., argon or nitrogen) to prevent hydrolysis . Use explosion-proof equipment and avoid exposure to heat, sparks, or open flames due to its low flash point (110°C) . Always handle in a chemical fume hood with appropriate PPE (gloves, goggles, face shield) .
Q. How is this reagent utilized in oligonucleotide synthesis, and what purity standards are required?
It acts as a phosphitylating agent in solid-phase DNA/RNA synthesis, activating nucleosides for phosphoramidite coupling . Purity ≥97% (by 31P-NMR) is critical to minimize side reactions . The reaction typically employs 1H-tetrazole as an activator, facilitating nucleophilic attack on the phosphorus center . Post-reaction, quenching with saturated NaHCO₃ and purification via column chromatography (ethyl acetate/hexane) are standard .
Q. What are the key physical properties influencing experimental design?
Physical state discrepancies exist: it is reported as a liquid (density 1.061 g/mL at 25°C) or crystalline powder , likely due to batch-specific impurities or storage conditions. The refractive index (1.476–1.480) and solubility in anhydrous CH₂Cl₂ make it suitable for low-temperature reactions . Avoid aqueous solvents due to rapid hydrolysis .
Advanced Research Questions
Q. How can reaction conditions be optimized for phosphitylation of sensitive substrates (e.g., unprotected nucleosides)?
Use anhydrous CH₂Cl₂ or acetonitrile with N,N-diisopropylethylamine (DIPEA) to neutralize HCl byproducts . For sterically hindered substrates, extend reaction times (up to 16 hours) and monitor via 31P-NMR to confirm completion . Diastereomeric products may require silica gel chromatography with gradient elution (30–80% ethyl acetate/hexane) .
Q. What strategies mitigate β-elimination of the cyanoethyl group during phosphitylation?
Avoid strong bases (e.g., DIPEA) in excess, as they promote cleavage of the β-cyanoethyl protecting group . Instead, use milder activators like 5-ethylthio-1H-tetrazole (ETT) and maintain reaction temperatures below 25°C . Post-synthesis, stabilize the product by immediate oxidation (e.g., iodine/water) to convert trivalent phosphorus to phosphate .
Q. How does thermal instability impact large-scale synthesis, and what precautions are necessary?
Heating above 110°C risks explosive decomposition . For exothermic reactions, use cooling baths (0–5°C) and controlled addition of reagents. Monitor temperature with in-line probes and avoid incompatible materials (strong oxidizers, alcohols) . Post-reaction, residues should be quenched with cold isopropanol and disposed per hazardous waste protocols .
Q. What analytical methods validate the compound’s purity and structural integrity?
31P-NMR is essential to confirm phosphoramidite formation (δ ~149 ppm) and detect hydrolyzed byproducts (e.g., H-phosphonates, δ ~0–10 ppm) . High-resolution mass spectrometry (HRMS) and elemental analysis further verify molecular weight and composition . For trace moisture analysis, Karl Fischer titration is recommended .
Q. Can this reagent be adapted for solid-phase synthesis of modified oligonucleotides (e.g., fluorinated or methylated)?
Yes. demonstrates its use in polymer-bound phosphitylating reagents for coupling unprotected nucleosides . For 2′-fluoro or 4′-C-methoxy modifications, pre-activate the nucleoside with 1H-tetrazole and use stoichiometric reagent ratios to avoid over-phosphitylation .
Q. Data Contradictions and Resolution
- Physical State Discrepancy : Reports of liquid vs. crystalline forms likely arise from purity variations (e.g., 97% vs. higher grades) or solvent residues . Consistently dry the compound under vacuum before use.
- Flash Point Consistency : All sources agree on 110°C , confirming the need for inert-atmosphere handling.
Comparaison Avec Des Composés Similaires
Comparison with Similar Phosphoramidite Reagents
Structural and Functional Analogs
2-Cyanoethyl N,N,N',N'-Tetraisopropylphosphoramidite
Unlike the diisopropyl variant, it requires activation by 4,5-dicyanoimidazole (DCI) for phosphitylation. This modification reduces undesired side reactions, particularly with guanine nucleobases, and improves yields in LNA synthesis (e.g., 90% yield vs. 60–70% for the diisopropyl variant) .
Methyl N,N-Diisopropylphosphoramidite
A simpler analog lacking the cyanoethyl group, this compound is less sterically hindered but more prone to hydrolysis. Its lower molecular weight (MW ~183) and reduced stability limit its use in high-purity oligonucleotide synthesis.
Reactivity and Efficiency
- Diisopropylchlorophosphoramidite :
- Tetraisopropylphosphoramidite with DCI :
Stability and Handling
The diisopropylchlorophosphoramidite’s instability necessitates stringent storage conditions, increasing operational costs compared to more stable analogs .
Key Research Findings
LNA Synthesis : The diisopropylchlorophosphoramidite produced LNA phosphoramidites in 60–70% yields with side products, while the tetraisopropyl/DCI system achieved >90% yields and higher purity .
Modified Nucleotides : In fluorinated uridine synthesis, the diisopropyl variant yielded 86% for compound 14 but only 41% for compound 15, highlighting structural dependency .
Safety : Incidents of decomposition during scale-up (e.g., gas release) underscore the need for controlled environments .
Propriétés
IUPAC Name |
3-[chloro-[di(propan-2-yl)amino]phosphanyl]oxypropanenitrile | |
---|---|---|
Source | PubChem | |
URL | https://pubchem.ncbi.nlm.nih.gov | |
Description | Data deposited in or computed by PubChem | |
InChI |
InChI=1S/C9H18ClN2OP/c1-8(2)12(9(3)4)14(10)13-7-5-6-11/h8-9H,5,7H2,1-4H3 | |
Source | PubChem | |
URL | https://pubchem.ncbi.nlm.nih.gov | |
Description | Data deposited in or computed by PubChem | |
InChI Key |
QWTBDIBOOIAZEF-UHFFFAOYSA-N | |
Source | PubChem | |
URL | https://pubchem.ncbi.nlm.nih.gov | |
Description | Data deposited in or computed by PubChem | |
Canonical SMILES |
CC(C)N(C(C)C)P(OCCC#N)Cl | |
Source | PubChem | |
URL | https://pubchem.ncbi.nlm.nih.gov | |
Description | Data deposited in or computed by PubChem | |
Molecular Formula |
C9H18ClN2OP | |
Source | PubChem | |
URL | https://pubchem.ncbi.nlm.nih.gov | |
Description | Data deposited in or computed by PubChem | |
DSSTOX Substance ID |
DTXSID60370534 | |
Record name | 2-Cyanoethyl N,N-diisopropylchlorophosphoramidite | |
Source | EPA DSSTox | |
URL | https://comptox.epa.gov/dashboard/DTXSID60370534 | |
Description | DSSTox provides a high quality public chemistry resource for supporting improved predictive toxicology. | |
Molecular Weight |
236.68 g/mol | |
Source | PubChem | |
URL | https://pubchem.ncbi.nlm.nih.gov | |
Description | Data deposited in or computed by PubChem | |
CAS No. |
89992-70-1 | |
Record name | 2-Cyanoethyl N,N-diisopropylchlorophosphoramidite | |
Source | CAS Common Chemistry | |
URL | https://commonchemistry.cas.org/detail?cas_rn=89992-70-1 | |
Description | CAS Common Chemistry is an open community resource for accessing chemical information. Nearly 500,000 chemical substances from CAS REGISTRY cover areas of community interest, including common and frequently regulated chemicals, and those relevant to high school and undergraduate chemistry classes. This chemical information, curated by our expert scientists, is provided in alignment with our mission as a division of the American Chemical Society. | |
Explanation | The data from CAS Common Chemistry is provided under a CC-BY-NC 4.0 license, unless otherwise stated. | |
Record name | 2-Cyanoethyl N,N-diisopropylchlorophosphoramidite | |
Source | EPA DSSTox | |
URL | https://comptox.epa.gov/dashboard/DTXSID60370534 | |
Description | DSSTox provides a high quality public chemistry resource for supporting improved predictive toxicology. | |
Record name | 2-Cyanoethyl diisopropylchlorophos phoramidite | |
Source | European Chemicals Agency (ECHA) | |
URL | https://echa.europa.eu/information-on-chemicals | |
Description | The European Chemicals Agency (ECHA) is an agency of the European Union which is the driving force among regulatory authorities in implementing the EU's groundbreaking chemicals legislation for the benefit of human health and the environment as well as for innovation and competitiveness. | |
Explanation | Use of the information, documents and data from the ECHA website is subject to the terms and conditions of this Legal Notice, and subject to other binding limitations provided for under applicable law, the information, documents and data made available on the ECHA website may be reproduced, distributed and/or used, totally or in part, for non-commercial purposes provided that ECHA is acknowledged as the source: "Source: European Chemicals Agency, http://echa.europa.eu/". Such acknowledgement must be included in each copy of the material. ECHA permits and encourages organisations and individuals to create links to the ECHA website under the following cumulative conditions: Links can only be made to webpages that provide a link to the Legal Notice page. | |
Synthesis routes and methods I
Procedure details
Synthesis routes and methods II
Procedure details
Avertissement et informations sur les produits de recherche in vitro
Veuillez noter que tous les articles et informations sur les produits présentés sur BenchChem sont destinés uniquement à des fins informatives. Les produits disponibles à l'achat sur BenchChem sont spécifiquement conçus pour des études in vitro, qui sont réalisées en dehors des organismes vivants. Les études in vitro, dérivées du terme latin "in verre", impliquent des expériences réalisées dans des environnements de laboratoire contrôlés à l'aide de cellules ou de tissus. Il est important de noter que ces produits ne sont pas classés comme médicaments et n'ont pas reçu l'approbation de la FDA pour la prévention, le traitement ou la guérison de toute condition médicale, affection ou maladie. Nous devons souligner que toute forme d'introduction corporelle de ces produits chez les humains ou les animaux est strictement interdite par la loi. Il est essentiel de respecter ces directives pour assurer la conformité aux normes légales et éthiques en matière de recherche et d'expérimentation.