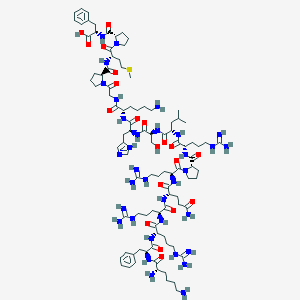
Précurseur d'Apeline (61-77) (humain, bovin, souris, rat) trifluoroacétate
- Cliquez sur DEMANDE RAPIDE pour recevoir un devis de notre équipe d'experts.
- Avec des produits de qualité à un prix COMPÉTITIF, vous pouvez vous concentrer davantage sur votre recherche.
Vue d'ensemble
Description
Apelin precursor (61-77) (human, bovine, mouse, rat) trifluoroacetate is a bioactive peptide derived from the apelin precursor protein. Apelin is an endogenous ligand for the G protein-coupled receptor APJ, which is involved in various physiological processes including cardiovascular regulation, fluid homeostasis, and energy metabolism . The apelin precursor protein consists of 77 amino acids and is cleaved by endopeptidases to generate several active forms, including apelin-12, apelin-13, apelin-17, and apelin-36 .
Applications De Recherche Scientifique
Apelin precursor (61-77) has a wide range of scientific research applications:
Chemistry: Used as a model peptide for studying peptide synthesis and modification techniques.
Biology: Investigated for its role in cellular signaling pathways and physiological processes.
Medicine: Explored as a potential therapeutic agent for cardiovascular diseases, metabolic disorders, and cancer.
Industry: Utilized in the development of peptide-based drugs and diagnostic tools.
Mécanisme D'action
Mode of Action
Apelin precursor (61-77) interacts with its target, the APJ receptor, to exert its effects. It has been found that apelins can increase the tolerance of the heart, brain, lung, and intestine to ischemia/reperfusion (I/R) . Protein kinases are involved in the neuroprotective, cardioprotective, renoprotective, and pulmonoprotective effects of apelins . Mitochondrial permeability transition pore and ATP-sensitive K+ channels are also involved in the protective effects of apelins .
Biochemical Pathways
The Apelin/APJ system affects multiple biochemical pathways. It inhibits apoptosis and ferroptosis, and activates autophagy of cardiomyocytes . It also increases vascular endothelial growth factor (VEGF) expression and decreases inducible NO-synthase activity .
Pharmacokinetics
It is known that the apelin precursor peptide is composed of 77 amino acid residues and is subsequently cleaved into different amino acid length fragments, including apelin-12, apelin-13, apelin-17, and apelin-36 . These peptides can be produced through peptide synthesis .
Result of Action
The action of Apelin precursor (61-77) results in increased tolerance of organs and cells to hypoxia and reoxygenation . It has been found to prevent reperfusion blood-brain barrier (BBB) injury, reduce the number of TUNEL-positive cells, and decrease inducible NO-synthase activity . These effects suggest that enzyme-resistant apelin analogues are promising peptides for the treatment of acute myocardial infarction (AMI), stroke, and I/R injury of lungs and kidneys .
Analyse Biochimique
Biochemical Properties
Apelin precursor (61-77) (human, bovine, mouse, rat) trifluoroacetate acts as an endogenous ligand for the G-protein-coupled receptor APJ . This interaction is pivotal in mediating the peptide’s effects on cardiovascular functions, such as enhancing myocardial contractility and promoting vasodilation . The peptide also interacts with various enzymes and proteins, including those involved in insulin secretion and pituitary hormone release . These interactions are essential for maintaining metabolic homeostasis and regulating fluid balance in the body .
Cellular Effects
Apelin precursor (61-77) (human, bovine, mouse, rat) trifluoroacetate exerts significant effects on different cell types and cellular processes. In endothelial cells, it promotes angiogenesis and enhances vascular permeability . In cardiomyocytes, the peptide improves contractility and protects against ischemic injury . Additionally, it influences cell signaling pathways, such as the PI3K/Akt and MAPK pathways, which are crucial for cell survival and proliferation . The peptide also modulates gene expression related to glucose metabolism and insulin sensitivity .
Molecular Mechanism
The molecular mechanism of action of apelin precursor (61-77) (human, bovine, mouse, rat) trifluoroacetate involves binding to the APJ receptor, which activates downstream signaling pathways . This binding leads to the activation of G-proteins, which in turn modulate various intracellular signaling cascades . The peptide can inhibit or activate enzymes, such as adenylate cyclase, affecting cyclic AMP levels and influencing cellular responses . Additionally, it can alter gene expression by modulating transcription factors involved in metabolic regulation .
Temporal Effects in Laboratory Settings
In laboratory settings, the effects of apelin precursor (61-77) (human, bovine, mouse, rat) trifluoroacetate can change over time. The peptide is relatively stable under physiological conditions but can degrade over extended periods . Long-term studies have shown that continuous exposure to the peptide can lead to sustained improvements in cardiovascular function and metabolic regulation . Degradation products may accumulate and potentially alter its biological activity .
Dosage Effects in Animal Models
The effects of apelin precursor (61-77) (human, bovine, mouse, rat) trifluoroacetate vary with different dosages in animal models. Low doses have been shown to enhance myocardial contractility and improve insulin sensitivity without adverse effects . Higher doses can lead to hypotension and potential toxicity . Threshold effects have been observed, where a minimum effective dose is required to achieve significant biological responses .
Metabolic Pathways
Apelin precursor (61-77) (human, bovine, mouse, rat) trifluoroacetate is involved in several metabolic pathways. It interacts with enzymes such as angiotensin-converting enzyme 2 (ACE2), which can degrade the peptide and modulate its activity . The peptide also influences metabolic flux by regulating glucose uptake and lipid metabolism . These interactions are crucial for maintaining energy balance and metabolic homeostasis .
Transport and Distribution
The transport and distribution of apelin precursor (61-77) (human, bovine, mouse, rat) trifluoroacetate within cells and tissues involve specific transporters and binding proteins . The peptide is widely distributed in various organs, including the heart, lungs, kidneys, and brain . It can accumulate in specific tissues, where it exerts its biological effects . The distribution pattern is influenced by factors such as receptor expression and tissue-specific transport mechanisms .
Subcellular Localization
Apelin precursor (61-77) (human, bovine, mouse, rat) trifluoroacetate is localized in specific subcellular compartments, which can affect its activity and function . The peptide is often found in the cytoplasm and can translocate to the nucleus under certain conditions . Post-translational modifications, such as phosphorylation, can influence its localization and interaction with other biomolecules . These modifications are essential for regulating the peptide’s biological activity and ensuring its proper function within the cell .
Méthodes De Préparation
Synthetic Routes and Reaction Conditions
The synthesis of apelin precursor (61-77) typically involves solid-phase peptide synthesis (SPPS), a method widely used for the production of peptides. The process begins with the attachment of the C-terminal amino acid to a solid resin, followed by the sequential addition of protected amino acids. Each amino acid is coupled using reagents such as N,N’-diisopropylcarbodiimide (DIC) and hydroxybenzotriazole (HOBt). After the peptide chain is assembled, it is cleaved from the resin and deprotected using trifluoroacetic acid (TFA), resulting in the formation of the trifluoroacetate salt .
Industrial Production Methods
Industrial production of apelin precursor (61-77) involves large-scale SPPS, utilizing automated peptide synthesizers to increase efficiency and yield. The process is optimized to ensure high purity and consistency of the final product. Quality control measures, such as high-performance liquid chromatography (HPLC) and mass spectrometry, are employed to verify the identity and purity of the synthesized peptide .
Analyse Des Réactions Chimiques
Types of Reactions
Apelin precursor (61-77) can undergo various chemical reactions, including:
Oxidation: The methionine residues in the peptide can be oxidized to methionine sulfoxide or methionine sulfone.
Reduction: Disulfide bonds, if present, can be reduced to free thiol groups using reducing agents like dithiothreitol (DTT).
Substitution: Amino acid residues can be substituted with analogs to study structure-activity relationships.
Common Reagents and Conditions
Oxidation: Hydrogen peroxide (H2O2) or performic acid can be used under mild conditions.
Reduction: DTT or tris(2-carboxyethyl)phosphine (TCEP) are commonly used reducing agents.
Substitution: Amino acid analogs are incorporated during SPPS using standard coupling reagents.
Major Products
The major products formed from these reactions include oxidized or reduced forms of the peptide, as well as analogs with substituted amino acids. These modifications can alter the peptide’s biological activity and stability .
Comparaison Avec Des Composés Similaires
Similar Compounds
Apelin-12: A shorter active form of apelin with distinct biological activity.
Apelin-13: Known for its strong activity and involvement in cardiovascular regulation.
Apelin-17: Another active form with unique signaling properties.
Apelin-36: The longest active form with different physiological effects.
Uniqueness
Apelin precursor (61-77) is unique due to its specific sequence and the presence of trifluoroacetate, which can influence its stability and solubility. Compared to other apelin forms, it may exhibit different binding affinities and signaling profiles, making it a valuable tool for studying the apelin/APJ system.
Propriétés
Numéro CAS |
217082-57-0 |
---|---|
Formule moléculaire |
C96H156N34O20S |
Poids moléculaire |
2138.6 g/mol |
Nom IUPAC |
(2S)-2-[[(2S)-1-[(2S)-2-[[(2S)-1-[2-[[(2S)-6-amino-2-[[(2S)-2-[[(2S)-2-[[(2S)-2-[[(2S)-2-[[(2S)-1-[(2S)-2-[[(2S)-5-amino-2-[[(2S)-5-carbamimidamido-2-[[(2S)-5-carbamimidamido-2-[[(2S)-2-[[(2S)-2,6-diaminohexanoyl]amino]-3-phenylpropanoyl]amino]pentanoyl]amino]pentanoyl]amino]-5-oxopentanoyl]amino]-5-carbamimidamidopentanoyl]pyrrolidine-2-carbonyl]amino]-5-carbamimidamidopentanoyl]amino]-4-methylpentanoyl]amino]-3-hydroxypropanoyl]amino]-3-(1H-imidazol-5-yl)propanoyl]amino]hexanoyl]amino]acetyl]pyrrolidine-2-carbonyl]amino]-4-methylsulfanylbutanoyl]pyrrolidine-2-carbonyl]amino]-3-phenylpropanoic acid |
InChI |
InChI=1S/C96H156N34O20S/c1-55(2)47-67(83(140)127-71(53-131)86(143)125-69(50-58-51-109-54-115-58)85(142)116-60(26-11-13-38-98)78(135)114-52-76(133)128-43-18-31-72(128)87(144)122-66(36-46-151-3)91(148)130-45-20-33-74(130)89(146)126-70(92(149)150)49-57-23-8-5-9-24-57)124-81(138)63(29-16-41-112-95(105)106)120-88(145)73-32-19-44-129(73)90(147)65(30-17-42-113-96(107)108)121-82(139)64(34-35-75(100)132)119-80(137)61(27-14-39-110-93(101)102)117-79(136)62(28-15-40-111-94(103)104)118-84(141)68(48-56-21-6-4-7-22-56)123-77(134)59(99)25-10-12-37-97/h4-9,21-24,51,54-55,59-74,131H,10-20,25-50,52-53,97-99H2,1-3H3,(H2,100,132)(H,109,115)(H,114,135)(H,116,142)(H,117,136)(H,118,141)(H,119,137)(H,120,145)(H,121,139)(H,122,144)(H,123,134)(H,124,138)(H,125,143)(H,126,146)(H,127,140)(H,149,150)(H4,101,102,110)(H4,103,104,111)(H4,105,106,112)(H4,107,108,113)/t59-,60-,61-,62-,63-,64-,65-,66-,67-,68-,69-,70-,71-,72-,73-,74-/m0/s1 |
Clé InChI |
SVWSKJCJNAIKNH-MJZUAXFLSA-N |
SMILES |
CC(C)CC(C(=O)NC(CO)C(=O)NC(CC1=CN=CN1)C(=O)NC(CCCCN)C(=O)NCC(=O)N2CCCC2C(=O)NC(CCSC)C(=O)N3CCCC3C(=O)NC(CC4=CC=CC=C4)C(=O)O)NC(=O)C(CCCNC(=N)N)NC(=O)C5CCCN5C(=O)C(CCCNC(=N)N)NC(=O)C(CCC(=O)N)NC(=O)C(CCCNC(=N)N)NC(=O)C(CCCNC(=N)N)NC(=O)C(CC6=CC=CC=C6)NC(=O)C(CCCCN)N |
SMILES isomérique |
CC(C)C[C@@H](C(=O)N[C@@H](CO)C(=O)N[C@@H](CC1=CN=CN1)C(=O)N[C@@H](CCCCN)C(=O)NCC(=O)N2CCC[C@H]2C(=O)N[C@@H](CCSC)C(=O)N3CCC[C@H]3C(=O)N[C@@H](CC4=CC=CC=C4)C(=O)O)NC(=O)[C@H](CCCNC(=N)N)NC(=O)[C@@H]5CCCN5C(=O)[C@H](CCCNC(=N)N)NC(=O)[C@H](CCC(=O)N)NC(=O)[C@H](CCCNC(=N)N)NC(=O)[C@H](CCCNC(=N)N)NC(=O)[C@H](CC6=CC=CC=C6)NC(=O)[C@H](CCCCN)N |
SMILES canonique |
CC(C)CC(C(=O)NC(CO)C(=O)NC(CC1=CN=CN1)C(=O)NC(CCCCN)C(=O)NCC(=O)N2CCCC2C(=O)NC(CCSC)C(=O)N3CCCC3C(=O)NC(CC4=CC=CC=C4)C(=O)O)NC(=O)C(CCCNC(=N)N)NC(=O)C5CCCN5C(=O)C(CCCNC(=N)N)NC(=O)C(CCC(=O)N)NC(=O)C(CCCNC(=N)N)NC(=O)C(CCCNC(=N)N)NC(=O)C(CC6=CC=CC=C6)NC(=O)C(CCCCN)N |
Séquence |
KFRRQRPRLSHKGPMPF |
Synonymes |
Alternative Names: K17F, Prepro-61-77-Apelin |
Origine du produit |
United States |
Avertissement et informations sur les produits de recherche in vitro
Veuillez noter que tous les articles et informations sur les produits présentés sur BenchChem sont destinés uniquement à des fins informatives. Les produits disponibles à l'achat sur BenchChem sont spécifiquement conçus pour des études in vitro, qui sont réalisées en dehors des organismes vivants. Les études in vitro, dérivées du terme latin "in verre", impliquent des expériences réalisées dans des environnements de laboratoire contrôlés à l'aide de cellules ou de tissus. Il est important de noter que ces produits ne sont pas classés comme médicaments et n'ont pas reçu l'approbation de la FDA pour la prévention, le traitement ou la guérison de toute condition médicale, affection ou maladie. Nous devons souligner que toute forme d'introduction corporelle de ces produits chez les humains ou les animaux est strictement interdite par la loi. Il est essentiel de respecter ces directives pour assurer la conformité aux normes légales et éthiques en matière de recherche et d'expérimentation.