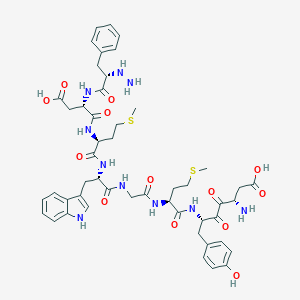
H-ASP-TYR-MET-GLY-TRP-MET-ASP-PHE-NH2
- Cliquez sur DEMANDE RAPIDE pour recevoir un devis de notre équipe d'experts.
- Avec des produits de qualité à un prix COMPÉTITIF, vous pouvez vous concentrer davantage sur votre recherche.
Vue d'ensemble
Description
Asp-Tyr-Met-Gly-Trp-Met-Asp-Phe-NH2 is an eight-membered oligopeptide consisting of Asp, Tyr, Met, Gly, Trp, Met, As and Phe-NH2 residues joined in sequence. It has a role as a human metabolite, a rat metabolite and a mouse metabolite. It is an oligopeptide and a peptidyl amide.
Applications De Recherche Scientifique
1. Régulation de la sécrétion du peptide natriurétique auriculaire (ANP) La cholecystokinine octapeptide (CCK-8) s'est avérée favoriser la sécrétion d'ANP par l'activation de la signalisation NOX4–PGC-1α–PPARα/PPARγ dans des oreillettes de rats isolées et battantes . L'ANP est une hormone cardiaque canonique qui est principalement sécrétée par les myocytes auriculaires et qui participe à la régulation des fluides corporels, à l'homéostasie de la pression artérielle et aux antioxydants .
Régulations cardiovasculaires
La CCK-8 se retrouve également dans les cardiomyocytes en tant que nouvelle hormone cardiaque et induit de multiples régulations cardiovasculaires . Elle exerce un effet inotrope négatif sur la dynamique mécanique auriculaire par l'activation des canaux potassiques sensibles à l'ATP et des canaux potassiques calcium-activés à grande conductance .
Amélioration de la synaptogenèse glutamatergique de l'hippocampe
La CCK-8 s'est avérée améliorer la synaptogenèse glutamatergique de l'hippocampe et la cognition postopératoire en inhibant l'induction des astrocytes réactifs A1 chez des souris âgées . Cela indique que la CCK-8 pourrait potentiellement être utilisée comme agent thérapeutique pour le rétablissement neurocognitif retardé (dNCR), une complication postopératoire fréquente chez les patients chirurgicaux gériatriques .
Suppression de l'activation de la microglie
L'administration de CCK-8 s'est avérée supprimer l'activation de la microglie, un type de cellule gliale située dans tout le cerveau et la moelle épinière .
5. Inhibition de l'induction des astrocytes réactifs A1 La CCK-8 s'est avérée inhiber l'induction des astrocytes réactifs A1, qui sont un sous-type d'astrocytes qui deviennent réactifs en réponse à une blessure .
Régulation du rétablissement neurocognitif
La CCK-8 favorise le rétablissement neurocognitif chez les souris âgées atteintes de rétablissement neurocognitif retardé (dNCR) après une intervention chirurgicale . Cela suggère que la CCK-8 pourrait potentiellement être utilisée pour améliorer la fonction cognitive chez les patients gériatriques après une intervention chirurgicale<a aria-label="2: 6. Régulation du rétablissement neurocognitif La CCK-8 favorise le rétablissement neurocognitif chez les souris âgées
Mécanisme D'action
Target of Action
The primary target of Cholecystokinin Octapeptide, desulfated, also known as CCK(26-33) Desulfated, is the Cholecystokinin Receptor (CCKR) . This receptor is a G-protein coupled receptor located in the gastrointestinal system and the central nervous system .
Mode of Action
CCK(26-33) Desulfated interacts with its target, the CCKR, to stimulate various physiological responses. It acts as an agonist at the receptor, meaning it binds to the receptor and activates it . This activation triggers a cascade of intracellular events, leading to the physiological responses associated with CCK, such as the release of digestive enzymes and the contraction of the gallbladder .
Biochemical Pathways
Upon activation of the CCKR by CCK(26-33) Desulfated, several biochemical pathways are affected. One of the key pathways is the Phospholipase C pathway , which leads to the production of inositol trisphosphate (IP3) and diacylglycerol (DAG). These molecules further trigger the release of calcium ions and the activation of protein kinase C, respectively . These events lead to various downstream effects, including the release of digestive enzymes and the contraction of the gallbladder .
Pharmacokinetics
As a peptide, it is expected to be absorbed in the gastrointestinal tract, distributed throughout the body, metabolized by various enzymes, and excreted via the kidneys .
Result of Action
The activation of the CCKR by CCK(26-33) Desulfated leads to several molecular and cellular effects. These include the release of digestive enzymes from the pancreas, contraction of the gallbladder, slowing of gastric emptying, and induction of satiety . These effects facilitate digestion and regulate food intake .
Action Environment
The action, efficacy, and stability of CCK(26-33) Desulfated can be influenced by various environmental factors. For instance, the presence of food in the stomach can enhance the release of CCK, thereby potentiating its effects . Additionally, the stability of CCK(26-33) Desulfated can be affected by pH and temperature, with extreme conditions potentially leading to degradation of the peptide .
Analyse Biochimique
Biochemical Properties
Cholecystokinin Octapeptide, desulfated, interacts with various enzymes, proteins, and other biomolecules in biochemical reactions. It acts both as a hormone and a neurotransmitter and is found in the gastrointestinal (GI) system and the central nervous system . It is a satiety peptide that inhibits food intake .
Cellular Effects
Cholecystokinin Octapeptide, desulfated, has significant effects on various types of cells and cellular processes. It influences cell function, including impacts on cell signaling pathways, gene expression, and cellular metabolism .
Propriétés
{ "Design of the Synthesis Pathway": "The compound 'Asp-Tyr-Met-Gly-Trp-Met-Asp-Phe-NH2' can be synthesized using solid-phase peptide synthesis (SPPS) method. SPPS involves the stepwise addition of protected amino acids to a solid support, followed by deprotection and cleavage from the support to obtain the desired peptide.", "Starting Materials": [ "Fmoc-Asp(OtBu)-OH", "Fmoc-Tyr(tBu)-OH", "Fmoc-Met(OtBu)-OH", "Fmoc-Gly-OH", "Fmoc-Trp(Boc)-OH", "Fmoc-Met(OtBu)-OH", "Fmoc-Asp(OtBu)-OH", "Fmoc-Phe-OH", "NH2-PEG-PS resin" ], "Reaction": [ "Step 1: Loading of NH2-PEG-PS resin onto the reaction vessel.", "Step 2: Deprotection of Fmoc group using 20% piperidine in DMF.", "Step 3: Coupling of Fmoc-Asp(OtBu)-OH using HATU/HOBT/DIPEA in DMF.", "Step 4: Deprotection of Fmoc group using 20% piperidine in DMF.", "Step 5: Coupling of Fmoc-Tyr(tBu)-OH using HATU/HOBT/DIPEA in DMF.", "Step 6: Deprotection of Fmoc group using 20% piperidine in DMF.", "Step 7: Coupling of Fmoc-Met(OtBu)-OH using HATU/HOBT/DIPEA in DMF.", "Step 8: Deprotection of Fmoc group using 20% piperidine in DMF.", "Step 9: Coupling of Fmoc-Gly-OH using HATU/HOBT/DIPEA in DMF.", "Step 10: Deprotection of Fmoc group using 20% piperidine in DMF.", "Step 11: Coupling of Fmoc-Trp(Boc)-OH using HATU/HOBT/DIPEA in DMF.", "Step 12: Deprotection of Fmoc group using 20% piperidine in DMF.", "Step 13: Coupling of Fmoc-Met(OtBu)-OH using HATU/HOBT/DIPEA in DMF.", "Step 14: Deprotection of Fmoc group using 20% piperidine in DMF.", "Step 15: Coupling of Fmoc-Asp(OtBu)-OH using HATU/HOBT/DIPEA in DMF.", "Step 16: Deprotection of Fmoc group using 20% piperidine in DMF.", "Step 17: Coupling of Fmoc-Phe-OH using HATU/HOBT/DIPEA in DMF.", "Step 18: Final deprotection and cleavage of the peptide from the resin using TFA/H2O/TIS.", "Step 19: Purification of the crude peptide by HPLC.", "Step 20: Characterization of the purified peptide by analytical HPLC and mass spectrometry." ] } | |
Numéro CAS |
25679-24-7 |
Formule moléculaire |
C49H62N10O13S2 |
Poids moléculaire |
1063.2 g/mol |
Nom IUPAC |
(3S,6S)-3-amino-6-[[(2S)-2-[[2-[[(2S)-2-[[(2S)-2-[[(2S)-3-carboxy-2-[[(2S)-2-hydrazinyl-3-phenylpropanoyl]amino]propanoyl]amino]-4-methylsulfanylbutanoyl]amino]-3-(1H-indol-3-yl)propanoyl]amino]acetyl]amino]-4-methylsulfanylbutanoyl]amino]-7-(4-hydroxyphenyl)-4,5-dioxoheptanoic acid |
InChI |
InChI=1S/C49H62N10O13S2/c1-73-18-16-34(46(69)56-36(20-28-12-14-30(60)15-13-28)44(67)43(66)32(50)23-41(62)63)54-40(61)26-53-45(68)37(22-29-25-52-33-11-7-6-10-31(29)33)57-47(70)35(17-19-74-2)55-48(71)38(24-42(64)65)58-49(72)39(59-51)21-27-8-4-3-5-9-27/h3-15,25,32,34-39,52,59-60H,16-24,26,50-51H2,1-2H3,(H,53,68)(H,54,61)(H,55,71)(H,56,69)(H,57,70)(H,58,72)(H,62,63)(H,64,65)/t32-,34-,35-,36-,37-,38-,39-/m0/s1 |
Clé InChI |
ZBTPHEHKAHBSMT-YRVFCXMDSA-N |
SMILES isomérique |
CSCC[C@@H](C(=O)N[C@@H](CC1=CC=C(C=C1)O)C(=O)C(=O)[C@H](CC(=O)O)N)NC(=O)CNC(=O)[C@H](CC2=CNC3=CC=CC=C32)NC(=O)[C@H](CCSC)NC(=O)[C@H](CC(=O)O)NC(=O)[C@H](CC4=CC=CC=C4)NN |
SMILES |
CSCCC(C(=O)NCC(=O)NC(CC1=CNC2=CC=CC=C21)C(=O)NC(CCSC)C(=O)NC(CC(=O)O)C(=O)NC(CC3=CC=CC=C3)C(=O)N)NC(=O)C(CC4=CC=C(C=C4)O)NC(=O)C(CC(=O)O)N |
SMILES canonique |
CSCCC(C(=O)NC(CC1=CC=C(C=C1)O)C(=O)C(=O)C(CC(=O)O)N)NC(=O)CNC(=O)C(CC2=CNC3=CC=CC=C32)NC(=O)C(CCSC)NC(=O)C(CC(=O)O)NC(=O)C(CC4=CC=CC=C4)NN |
Séquence |
DYMGWMDF |
Synonymes |
des(SO3)CCK-8 desulfated cholecystokinin-8 desulfated sincalide |
Origine du produit |
United States |
Retrosynthesis Analysis
AI-Powered Synthesis Planning: Our tool employs the Template_relevance Pistachio, Template_relevance Bkms_metabolic, Template_relevance Pistachio_ringbreaker, Template_relevance Reaxys, Template_relevance Reaxys_biocatalysis model, leveraging a vast database of chemical reactions to predict feasible synthetic routes.
One-Step Synthesis Focus: Specifically designed for one-step synthesis, it provides concise and direct routes for your target compounds, streamlining the synthesis process.
Accurate Predictions: Utilizing the extensive PISTACHIO, BKMS_METABOLIC, PISTACHIO_RINGBREAKER, REAXYS, REAXYS_BIOCATALYSIS database, our tool offers high-accuracy predictions, reflecting the latest in chemical research and data.
Strategy Settings
Precursor scoring | Relevance Heuristic |
---|---|
Min. plausibility | 0.01 |
Model | Template_relevance |
Template Set | Pistachio/Bkms_metabolic/Pistachio_ringbreaker/Reaxys/Reaxys_biocatalysis |
Top-N result to add to graph | 6 |
Feasible Synthetic Routes
Avertissement et informations sur les produits de recherche in vitro
Veuillez noter que tous les articles et informations sur les produits présentés sur BenchChem sont destinés uniquement à des fins informatives. Les produits disponibles à l'achat sur BenchChem sont spécifiquement conçus pour des études in vitro, qui sont réalisées en dehors des organismes vivants. Les études in vitro, dérivées du terme latin "in verre", impliquent des expériences réalisées dans des environnements de laboratoire contrôlés à l'aide de cellules ou de tissus. Il est important de noter que ces produits ne sont pas classés comme médicaments et n'ont pas reçu l'approbation de la FDA pour la prévention, le traitement ou la guérison de toute condition médicale, affection ou maladie. Nous devons souligner que toute forme d'introduction corporelle de ces produits chez les humains ou les animaux est strictement interdite par la loi. Il est essentiel de respecter ces directives pour assurer la conformité aux normes légales et éthiques en matière de recherche et d'expérimentation.