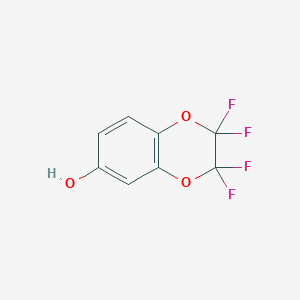
6-Hydroxy-2,2,3,3-tétrafluoro-1,4-benzodioxane
- Cliquez sur DEMANDE RAPIDE pour recevoir un devis de notre équipe d'experts.
- Avec des produits de qualité à un prix COMPÉTITIF, vous pouvez vous concentrer davantage sur votre recherche.
Vue d'ensemble
Description
L'azlocilline, sel de sodium, est un antibiotique pénicilline semisynthétique dérivé de l'ampicilline. Elle appartient à la classe des pénicillines acylureido et présente un large spectre d'activité antibactérienne. L'azlocilline, sel de sodium, est particulièrement efficace contre les bactéries à Gram négatif, y compris Pseudomonas aeruginosa, et présente également une activité contre certaines bactéries à Gram positif .
Applications De Recherche Scientifique
Azlocillin sodium salt has a wide range of scientific research applications, including:
Chemistry: Used as a model compound for studying β-lactam antibiotics and their interactions with bacterial enzymes.
Biology: Employed in microbiological studies to investigate the antibacterial activity against various bacterial strains.
Medicine: Utilized in clinical research to develop new antibiotic therapies and to study the pharmacokinetics and pharmacodynamics of β-lactam antibiotics.
Industry: Applied in the development of novel antibiotic quantitation techniques and in the production of high-purity antibiotic formulations
Méthodes De Préparation
Voies de synthèse et conditions réactionnelles
La synthèse de l'azlocilline, sel de sodium, implique l'activation d'un analogue de phénylglycine substitué avec du chlorure de 1,3-diméthyl-2-chloro-1-imidazolinium, suivie d'une condensation avec l'acide 6-aminopénicillanique . Les conditions réactionnelles comprennent généralement le maintien d'une température et d'un pH contrôlés pour garantir un rendement et une pureté optimaux.
Méthodes de production industrielle
Dans les milieux industriels, l'azlocilline, sel de sodium, est produite en soumettant l'acide azlocilline à une réaction de formation de sel, suivie d'une lyophilisation pour obtenir un produit stable et de haute pureté. Le procédé implique la préparation d'une solution d'agent de formation de sel de sodium, le refroidissement de la solution d'acide azlocilline et l'ajout de l'agent de formation de sel de sodium dans des conditions contrôlées. La solution résultante est ensuite filtrée, dégermée et lyophilisée pour produire de l'azlocilline, sel de sodium .
Analyse Des Réactions Chimiques
Types de réactions
L'azlocilline, sel de sodium, subit diverses réactions chimiques, notamment :
Oxydation : L'azlocilline peut être oxydée dans des conditions spécifiques, conduisant à la formation de dérivés oxydés.
Réduction : Les réactions de réduction peuvent modifier les groupes fonctionnels de la molécule d'azlocilline.
Substitution : L'azlocilline peut participer à des réactions de substitution, où les groupes fonctionnels sont remplacés par d'autres groupes.
Réactifs et conditions courants
Oxydation : Les oxydants courants comprennent le peroxyde d'hydrogène et le permanganate de potassium.
Réduction : Des agents réducteurs tels que le borohydrure de sodium et l'hydrure de lithium et d'aluminium sont utilisés.
Substitution : Divers nucléophiles et électrophiles peuvent être utilisés dans les réactions de substitution, en fonction du produit souhaité.
Principaux produits formés
Les principaux produits formés à partir de ces réactions dépendent des réactifs et des conditions spécifiques utilisés. Par exemple, l'oxydation peut produire des dérivés d'azlocilline oxydée, tandis que la réduction peut produire des formes réduites d'azlocilline.
Applications de la recherche scientifique
L'azlocilline, sel de sodium, a un large éventail d'applications dans la recherche scientifique, notamment :
Chimie : Utilisée comme composé modèle pour étudier les antibiotiques β-lactamines et leurs interactions avec les enzymes bactériennes.
Biologie : Employée dans des études microbiologiques pour étudier l'activité antibactérienne contre diverses souches bactériennes.
Médecine : Utilisée dans la recherche clinique pour développer de nouvelles thérapies antibiotiques et pour étudier la pharmacocinétique et la pharmacodynamie des antibiotiques β-lactamines.
Industrie : Appliquée dans le développement de nouvelles techniques de quantification des antibiotiques et dans la production de formulations d'antibiotiques de haute pureté
Mécanisme d'action
L'azlocilline, sel de sodium, exerce ses effets antibactériens en se liant à des protéines de liaison à la pénicilline (PBP) spécifiques situées à l'intérieur de la paroi cellulaire bactérienne. Cette liaison inhibe la troisième et dernière étape de la synthèse de la paroi cellulaire bactérienne, conduisant à la lyse cellulaire. La lyse est médiée par des enzymes autolytiques de la paroi cellulaire bactérienne, telles que les autolysines. L'azlocilline peut également interférer avec les inhibiteurs d'autolysines, renforçant ainsi son activité bactéricide .
Mécanisme D'action
Azlocillin sodium salt exerts its antibacterial effects by binding to specific penicillin-binding proteins (PBPs) located inside the bacterial cell wall. This binding inhibits the third and final stage of bacterial cell wall synthesis, leading to cell lysis. The lysis is mediated by bacterial cell wall autolytic enzymes such as autolysins. Azlocillin may also interfere with autolysin inhibitors, enhancing its bactericidal activity .
Comparaison Avec Des Composés Similaires
L'azlocilline, sel de sodium, est similaire à d'autres pénicillines acylureido telles que la mézlocilline et la pipéracilline. Elle présente un spectre d'activité plus large et une puissance in vitro plus élevée par rapport aux pénicillines carboxy. Les caractéristiques structurelles uniques de l'azlocilline contribuent à son activité antibactérienne accrue contre un large éventail de bactéries .
Liste des composés similaires
- Mézlocilline
- Pipéracilline
- Carbenicilline
- Ticarcilline
L'azlocilline, sel de sodium, se distingue par son efficacité contre les bactéries à Gram positif et à Gram négatif, y compris Pseudomonas aeruginosa et les entérocoques, ce qui en fait un antibiotique précieux dans les milieux cliniques et de recherche .
Propriétés
IUPAC Name |
2,2,3,3-tetrafluoro-1,4-benzodioxin-6-ol |
Source
|
---|---|---|
Source | PubChem | |
URL | https://pubchem.ncbi.nlm.nih.gov | |
Description | Data deposited in or computed by PubChem | |
InChI |
InChI=1S/C8H4F4O3/c9-7(10)8(11,12)15-6-3-4(13)1-2-5(6)14-7/h1-3,13H |
Source
|
Source | PubChem | |
URL | https://pubchem.ncbi.nlm.nih.gov | |
Description | Data deposited in or computed by PubChem | |
InChI Key |
QMCVHTQWRXVSMQ-UHFFFAOYSA-N |
Source
|
Source | PubChem | |
URL | https://pubchem.ncbi.nlm.nih.gov | |
Description | Data deposited in or computed by PubChem | |
Canonical SMILES |
C1=CC2=C(C=C1O)OC(C(O2)(F)F)(F)F |
Source
|
Source | PubChem | |
URL | https://pubchem.ncbi.nlm.nih.gov | |
Description | Data deposited in or computed by PubChem | |
Molecular Formula |
C8H4F4O3 |
Source
|
Source | PubChem | |
URL | https://pubchem.ncbi.nlm.nih.gov | |
Description | Data deposited in or computed by PubChem | |
Molecular Weight |
224.11 g/mol |
Source
|
Source | PubChem | |
URL | https://pubchem.ncbi.nlm.nih.gov | |
Description | Data deposited in or computed by PubChem | |
Avertissement et informations sur les produits de recherche in vitro
Veuillez noter que tous les articles et informations sur les produits présentés sur BenchChem sont destinés uniquement à des fins informatives. Les produits disponibles à l'achat sur BenchChem sont spécifiquement conçus pour des études in vitro, qui sont réalisées en dehors des organismes vivants. Les études in vitro, dérivées du terme latin "in verre", impliquent des expériences réalisées dans des environnements de laboratoire contrôlés à l'aide de cellules ou de tissus. Il est important de noter que ces produits ne sont pas classés comme médicaments et n'ont pas reçu l'approbation de la FDA pour la prévention, le traitement ou la guérison de toute condition médicale, affection ou maladie. Nous devons souligner que toute forme d'introduction corporelle de ces produits chez les humains ou les animaux est strictement interdite par la loi. Il est essentiel de respecter ces directives pour assurer la conformité aux normes légales et éthiques en matière de recherche et d'expérimentation.