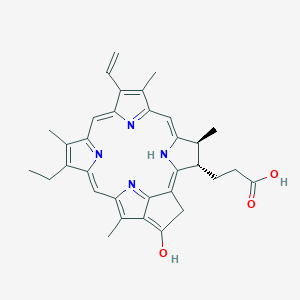
Pyropheophorbide a
Vue d'ensemble
Description
Le Pyropheophorbide-a est un dérivé de la chlorophylle qui a suscité un intérêt considérable en raison de ses propriétés uniques et de ses applications potentielles. Il s'agit d'un photosensibilisateur rouge foncé, ce qui signifie qu'il peut absorber la lumière et la convertir en énergie chimique. Ce composé est particulièrement connu pour son rôle dans la thérapie photodynamique, une modalité de traitement du cancer et d'autres maladies .
Applications De Recherche Scientifique
Pyropheophorbide-a has a wide range of scientific research applications, including:
Biology: It is used in studies of photosynthesis and chlorophyll metabolism.
Medicine: Pyropheophorbide-a is used in photodynamic therapy for the treatment of cancer and other diseases. .
Industry: It is used in the development of new materials and technologies, including solar cells and light-emitting devices
Mécanisme D'action
- PPB a activates several pathways:
Target of Action
Mode of Action
Biochemical Pathways
Pharmacokinetics (ADME)
Result of Action
Action Environment
Analyse Biochimique
Biochemical Properties
Pyropheophorbide a plays a significant role in biochemical reactions. It interacts with various enzymes, proteins, and other biomolecules. For instance, it is involved in the demethylation process catalyzed by an enzyme called pheophorbidase . This enzyme converts pheophorbide a to a precursor of this compound, C-13 2 -carboxylthis compound .
Cellular Effects
This compound has been shown to have significant effects on various types of cells. In photodynamic therapy, it can inhibit cell viability in a concentration- and light dose-dependent manner, and induce apoptosis via the mitochondrial apoptosis pathway . Additionally, it can induce autophagy in cells .
Molecular Mechanism
The mechanism of action of this compound involves the generation of reactive oxygen species (ROS) upon light exposure . When this compound interacts with light, it absorbs the energy and generates ROS, which subsequently react with cellular components, resulting in cell death .
Temporal Effects in Laboratory Settings
The effects of this compound change over time in laboratory settings. For instance, it has been shown to have a potent inhibitory effect on colorectal cancer stem cell-derived xenograft tumors in nude mice . This suggests that this compound has long-term effects on cellular function.
Dosage Effects in Animal Models
The effects of this compound vary with different dosages in animal models. For example, a study showed that porphysome nanoparticles, which include this compound, demonstrated significant tumor ablative effects at all doses tested, with the greatest effect seen with 10 mg/kg this compound at a drug-light interval of 24 hours .
Metabolic Pathways
This compound is involved in the chlorophyll degradation pathway in higher plants and algae . The enzyme pheophorbidase catalyzes the conversion of pheophorbide a to a precursor of this compound, C-13 2 -carboxylthis compound, by demethylation .
Transport and Distribution
It has been suggested that this compound can be transported and distributed within cells via endocytosis .
Subcellular Localization
It has been suggested that this compound can be localized in the mitochondria, where it induces apoptosis via the mitochondrial apoptosis pathway .
Méthodes De Préparation
Voies de synthèse et conditions de réaction : Le Pyropheophorbide-a peut être synthétisé par la dégradation de la chlorophylle-a. Le processus implique l'élimination de l'ion magnésium central et de la queue de phytol de la chlorophylle-a, suivie de la formation de pyropheophorbide-a. Cela peut être réalisé par différentes réactions chimiques, notamment l'hydrolyse acide et les réactions enzymatiques .
Méthodes de production industrielle : En milieu industriel, le pyropheophorbide-a est souvent produit à l'aide de techniques d'extraction et de purification à grande échelle. Le processus implique généralement l'extraction de la chlorophylle à partir de matières végétales, suivie d'une modification chimique pour obtenir du pyropheophorbide-a. L'utilisation de techniques chromatographiques avancées garantit la pureté et la qualité du produit final .
Analyse Des Réactions Chimiques
Types de réactions : Le Pyropheophorbide-a subit plusieurs types de réactions chimiques, notamment :
Oxydation : Cette réaction implique l'addition d'oxygène ou l'élimination d'hydrogène. Le pyropheophorbide-a peut être oxydé pour former divers dérivés.
Réduction : Cette réaction implique l'addition d'hydrogène ou l'élimination d'oxygène. Les réactions de réduction peuvent modifier la structure du pyropheophorbide-a, conduisant à différents produits.
Substitution : Cette réaction implique le remplacement d'un atome ou d'un groupe d'atomes par un autre. .
Réactifs et conditions courants :
Oxydation : Les oxydants courants comprennent le peroxyde d'hydrogène et le permanganate de potassium.
Réduction : Les réducteurs courants comprennent le borohydrure de sodium et l'hydrure de lithium et d'aluminium.
Substitution : Les réactifs courants comprennent les halogènes et les agents alkylants
Principaux produits formés :
4. Applications de la recherche scientifique
Le Pyropheophorbide-a a un large éventail d'applications en recherche scientifique, notamment :
Biologie : Il est utilisé dans les études de la photosynthèse et du métabolisme de la chlorophylle.
Médecine : Le Pyropheophorbide-a est utilisé en thérapie photodynamique pour le traitement du cancer et d'autres maladies. .
Industrie : Il est utilisé dans le développement de nouveaux matériaux et technologies, notamment les cellules solaires et les dispositifs émetteurs de lumière
5. Mécanisme d'action
Le mécanisme d'action du pyropheophorbide-a implique sa capacité à générer des espèces réactives de l'oxygène lors de l'irradiation par la lumière. Lorsqu'il est exposé à la lumière, le pyropheophorbide-a absorbe l'énergie et la transfère à l'oxygène moléculaire, produisant de l'oxygène singulet et d'autres espèces réactives de l'oxygène. Ces espèces réactives peuvent endommager les structures cellulaires, conduisant à la mort cellulaire. Ce mécanisme est particulièrement efficace en thérapie photodynamique, où le pyropheophorbide-a s'accumule sélectivement dans les cellules cancéreuses et les détruit lors de l'activation par la lumière .
Composés similaires :
Phéophorbide-a : Un autre dérivé de la chlorophylle présentant des propriétés photosensibilisatrices similaires.
Pyropheophorbide-a méthylique : Un dérivé méthylé du pyropheophorbide-a avec une solubilité et une activité photodynamique améliorées.
Pyropheophorbide-a de zinc : Un complexe de zinc du pyropheophorbide-a utilisé comme photosensibilisateur dans diverses applications.
Unicité : Le Pyropheophorbide-a est unique en raison de son rendement quantique élevé en oxygène singulet et de sa capacité à générer efficacement des espèces réactives de l'oxygène. Cela le rend particulièrement efficace en thérapie photodynamique et dans d'autres applications où des réactions induites par la lumière sont nécessaires .
Comparaison Avec Des Composés Similaires
Pheophorbide-a: Another chlorophyll derivative with similar photosensitizing properties.
Methyl pyropheophorbide-a: A methylated derivative of pyropheophorbide-a with enhanced solubility and photodynamic activity.
Zinc pyropheophorbide-a: A zinc complex of pyropheophorbide-a used as a photosensitizer in various applications.
Uniqueness: Pyropheophorbide-a is unique due to its high singlet oxygen quantum yield and its ability to generate reactive oxygen species efficiently. This makes it particularly effective in photodynamic therapy and other applications where light-induced reactions are required .
Propriétés
{ "Design of the Synthesis Pathway": "The synthesis of Pyropheophorbide a can be achieved through the modification of chlorophyll a. The process involves the removal of the magnesium ion from the chlorophyll a molecule, followed by oxidation and reduction reactions to produce Pyropheophorbide a.", "Starting Materials": [ "Chlorophyll a", "Acetic acid", "Methanol", "Hydrogen peroxide", "Sodium hydroxide", "Sodium dithionite", "Ethanol" ], "Reaction": [ "Chlorophyll a is dissolved in acetic acid and methanol.", "Hydrogen peroxide is added to the solution to oxidize the chlorophyll a.", "The solution is then heated to remove the magnesium ion, producing Pheophytin a.", "Sodium hydroxide is added to the solution to adjust the pH.", "Sodium dithionite is added to the solution to reduce the Pheophytin a to Pyropheophorbide a.", "The Pyropheophorbide a is then extracted from the solution using ethanol." ] } | |
Numéro CAS |
24533-72-0 |
Formule moléculaire |
C33H34N4O3 |
Poids moléculaire |
534.6 g/mol |
Nom IUPAC |
3-[(21S)-16-ethenyl-11-ethyl-4-hydroxy-12,17,21,26-tetramethyl-7,23,24,25-tetrazahexacyclo[18.2.1.15,8.110,13.115,18.02,6]hexacosa-1,4,6,8(26),9,11,13(25),14,16,18(24),19-undecaen-22-yl]propanoic acid |
InChI |
InChI=1S/C33H34N4O3/c1-7-19-15(3)23-12-25-17(5)21(9-10-30(39)40)32(36-25)22-11-29(38)31-18(6)26(37-33(22)31)14-28-20(8-2)16(4)24(35-28)13-27(19)34-23/h7,12-14,17,21,36,38H,1,8-11H2,2-6H3,(H,39,40)/t17-,21?/m0/s1 |
Clé InChI |
FDKRLXBXYZKWRZ-PBVYKCSPSA-N |
SMILES isomérique |
CCC1=C(C2=NC1=CC3=C(C4=C(CC(=C5C([C@@H](C(=CC6=NC(=C2)C(=C6C)C=C)N5)C)CCC(=O)O)C4=N3)O)C)C |
SMILES |
CCC1=C(C2=NC1=CC3=C(C4=C(CC(=C5C(C(C(=CC6=NC(=C2)C(=C6C)C=C)N5)C)CCC(=O)O)C4=N3)O)C)C |
SMILES canonique |
CCC1=C(C2=NC1=CC3=C(C4=C(CC(=C5C(C(C(=CC6=NC(=C2)C(=C6C)C=C)N5)C)CCC(=O)O)C4=N3)O)C)C |
Apparence |
A crystalline solid |
Pictogrammes |
Irritant |
Synonymes |
Pyrophaeophorbid A; (17S,18S)-3-Vinyl-3-deethyl-17,18-dihydrophytoporphyrin; 31,32-Didehydrophytochlorin; 3-Deethyl-3-vinylphytochlorin; Pyropheophorbide-alpha; Pyropheophorbide-α |
Origine du produit |
United States |
Retrosynthesis Analysis
AI-Powered Synthesis Planning: Our tool employs the Template_relevance Pistachio, Template_relevance Bkms_metabolic, Template_relevance Pistachio_ringbreaker, Template_relevance Reaxys, Template_relevance Reaxys_biocatalysis model, leveraging a vast database of chemical reactions to predict feasible synthetic routes.
One-Step Synthesis Focus: Specifically designed for one-step synthesis, it provides concise and direct routes for your target compounds, streamlining the synthesis process.
Accurate Predictions: Utilizing the extensive PISTACHIO, BKMS_METABOLIC, PISTACHIO_RINGBREAKER, REAXYS, REAXYS_BIOCATALYSIS database, our tool offers high-accuracy predictions, reflecting the latest in chemical research and data.
Strategy Settings
Precursor scoring | Relevance Heuristic |
---|---|
Min. plausibility | 0.01 |
Model | Template_relevance |
Template Set | Pistachio/Bkms_metabolic/Pistachio_ringbreaker/Reaxys/Reaxys_biocatalysis |
Top-N result to add to graph | 6 |
Feasible Synthetic Routes
Avertissement et informations sur les produits de recherche in vitro
Veuillez noter que tous les articles et informations sur les produits présentés sur BenchChem sont destinés uniquement à des fins informatives. Les produits disponibles à l'achat sur BenchChem sont spécifiquement conçus pour des études in vitro, qui sont réalisées en dehors des organismes vivants. Les études in vitro, dérivées du terme latin "in verre", impliquent des expériences réalisées dans des environnements de laboratoire contrôlés à l'aide de cellules ou de tissus. Il est important de noter que ces produits ne sont pas classés comme médicaments et n'ont pas reçu l'approbation de la FDA pour la prévention, le traitement ou la guérison de toute condition médicale, affection ou maladie. Nous devons souligner que toute forme d'introduction corporelle de ces produits chez les humains ou les animaux est strictement interdite par la loi. Il est essentiel de respecter ces directives pour assurer la conformité aux normes légales et éthiques en matière de recherche et d'expérimentation.