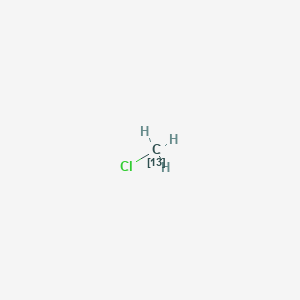
Chloromethane-13C
Vue d'ensemble
Description
Il est principalement utilisé comme agent antiviral dans les solutions ophtalmiques pour le traitement de la kératoconjonctivite et de la kératite épithéliale causées par les virus herpès simplex de type 1 et 2 . De plus, la trifluridine est un composant du médicament anticancéreux trifluridine/tipiracil, qui est utilisé dans le traitement du cancer colorectal métastatique .
Méthodes De Préparation
Voies de synthèse et conditions de réaction
La synthèse de la trifluridine implique plusieurs étapes clés :
Halogénation du ribose entièrement protégé par des hydroxyles : Cette étape implique l’halogénation du ribose entièrement protégé par des hydroxyles.
Condensation avec le 5-trifluorométhyl uracile : Le ribose halogéné est ensuite condensé avec le 5-trifluorométhyl uracile.
Déprotection : La 5-trifluorométhyl uridine intermédiaire est générée par déprotection du produit condensé.
Déshydratation, halogénation et réduction : Enfin, l’intermédiaire subit des réactions de déshydratation, d’halogénation et de réduction pour obtenir la trifluridine.
Méthodes de production industrielle
En production industrielle, la trifluridine est synthétisée en utilisant une méthode similaire, mais avec des optimisations pour la production à grande échelle. Par exemple, l’utilisation d’un catalyseur à base de résine acide au lieu d’un catalyseur acide de Lewis traditionnel améliore l’efficacité catalytique et réduit la pollution environnementale .
Analyse Des Réactions Chimiques
Types de réactions
La trifluridine subit diverses réactions chimiques, notamment :
Oxydation : La trifluridine peut être oxydée pour former divers produits d’oxydation.
Réduction : Les réactions de réduction peuvent convertir la trifluridine en différentes formes réduites.
Substitution : La trifluridine peut subir des réactions de substitution, en particulier impliquant son groupe trifluorométhyle.
Réactifs et conditions courantes
Oxydation : Les agents oxydants courants comprennent le peroxyde d’hydrogène et le permanganate de potassium.
Réduction : Des agents réducteurs tels que le borohydrure de sodium et l’hydrure de lithium et d’aluminium sont utilisés.
Substitution : Les réactions de substitution impliquent souvent des nucléophiles comme l’ammoniac ou les amines.
Principaux produits formés
Les principaux produits formés à partir de ces réactions comprennent divers dérivés de la trifluridine, tels que les dérivés de l’uracile substitués par un trifluorométhyle .
Applications de la recherche scientifique
La trifluridine a un large éventail d’applications de recherche scientifique :
Chimie : En chimie, la trifluridine est utilisée comme analogue de nucléoside dans diverses applications synthétiques et analytiques.
Biologie : En recherche biologique, la trifluridine est utilisée pour étudier les mécanismes de réplication et de réparation de l’ADN.
Médecine : La trifluridine est utilisée dans le traitement des infections virales, en particulier des infections à virus herpès simplex, et comme composant des thérapies anticancéreuses pour le cancer colorectal métastatique
Applications De Recherche Scientifique
Trifluridine has a wide range of scientific research applications:
Chemistry: In chemistry, trifluridine is used as a nucleoside analog in various synthetic and analytical applications.
Biology: In biological research, trifluridine is used to study DNA replication and repair mechanisms.
Medicine: Trifluridine is used in the treatment of viral infections, particularly herpes simplex virus infections, and as a component of anti-cancer therapies for metastatic colorectal cancer
Mécanisme D'action
La trifluridine exerce ses effets en s’incorporant à l’ADN et en interférant avec la synthèse de l’ADN. Il s’agit d’un analogue de nucléoside à base de thymidine qui est phosphorylé en triphosphate de trifluridine dans la cellule. Cette forme triphosphate est ensuite incorporée à l’ADN, conduisant à l’inhibition de la synthèse de l’ADN et de la prolifération cellulaire . Dans le cas de son activité antivirale, la trifluridine bloque la réplication de l’ADN viral en s’incorporant à l’ADN viral et en empêchant l’appariement de bases correct .
Comparaison Avec Des Composés Similaires
La trifluridine est souvent comparée à d’autres analogues de nucléosides tels que l’idoxuridine et la vidarabine. Bien que les trois composés soient utilisés pour traiter les infections virales, la trifluridine a montré une efficacité supérieure dans certains cas, en particulier dans le traitement des ulcères dendritiques causés par le virus herpès simplex . De plus, l’incorporation de la trifluridine à l’ADN et sa capacité à inhiber la thymidylate synthase la rendent unique parmi les analogues de nucléosides .
Liste des composés similaires
Idoxuridine : Un autre analogue de nucléoside utilisé pour traiter les infections à virus herpès simplex.
Vidarabine : Un médicament antiviral utilisé pour traiter les infections à virus herpès simplex et varicelle-zona.
Acyclovir : Un médicament antiviral largement utilisé pour les infections à virus herpès simplex et varicelle-zona.
Activité Biologique
Chloromethane-13C (CH3Cl-13C) is a stable isotope-labeled compound that has garnered interest in various fields, particularly in environmental science and microbiology. This article delves into its biological activity, focusing on its metabolic pathways, ecological impact, and implications for microbial communities.
Chloromethane, a simple alkyl halide, is naturally produced in the environment, primarily through biological processes in certain plants and microbial communities. The introduction of the 13C isotope allows for enhanced tracking of its metabolic pathways in biological systems.
Metabolic Pathways
-
Microbial Degradation :
- Certain bacteria can utilize chloromethane as a carbon source. For instance, studies have shown that members of the Alphaproteobacteria and Actinobacteria phyla exhibit significant chloromethane degradation capabilities when supplemented with methanol (CH3OH) as an additional substrate .
- A notable organism, Methylobacterium extorquens, although well-studied for its chloromethane degradation, was not found to be involved in certain soil sink activities, indicating a diverse microbial community responsible for this process .
-
Stable Isotope Labeling :
- The use of CH3Cl-13C in experiments allows researchers to trace the incorporation of carbon into microbial biomass and metabolites. This technique has revealed that chloromethane can be metabolized through pathways involving methanol and other one-carbon compounds, enhancing our understanding of microbial ecology in forest soils .
Ecological Impact
Chloromethane plays a crucial role in the global carbon cycle, particularly in forest ecosystems where it is produced and consumed by various microbial communities. Its degradation contributes to the removal of reactive chlorine species from the atmosphere, thus influencing atmospheric chemistry.
Table 1: Sources and Sinks of Chloromethane
Source/Sink | Description | Estimated Contribution |
---|---|---|
Natural Production | Emitted by tropical rainforests | 400-1100 Gg/year |
Microbial Degradation | Bacterial consumption in soils | Significant sink |
Atmospheric Reaction | Reacts with hydroxyl radicals (OH) | Major loss pathway |
Case Studies and Research Findings
-
Microcosm Experiments :
- In laboratory settings, microcosms were created to study the degradation rates of chloromethane in soil. These studies employed stable isotope probing (SIP) techniques to monitor changes in microbial community composition and activity over time .
- Results indicated that the presence of methanol significantly increased the chloromethane consumption rate, suggesting a competitive advantage for methylotrophic bacteria that can utilize multiple one-carbon substrates .
- Field Studies :
Propriétés
IUPAC Name |
chloro(113C)methane | |
---|---|---|
Source | PubChem | |
URL | https://pubchem.ncbi.nlm.nih.gov | |
Description | Data deposited in or computed by PubChem | |
InChI |
InChI=1S/CH3Cl/c1-2/h1H3/i1+1 | |
Source | PubChem | |
URL | https://pubchem.ncbi.nlm.nih.gov | |
Description | Data deposited in or computed by PubChem | |
InChI Key |
NEHMKBQYUWJMIP-OUBTZVSYSA-N | |
Source | PubChem | |
URL | https://pubchem.ncbi.nlm.nih.gov | |
Description | Data deposited in or computed by PubChem | |
Canonical SMILES |
CCl | |
Source | PubChem | |
URL | https://pubchem.ncbi.nlm.nih.gov | |
Description | Data deposited in or computed by PubChem | |
Isomeric SMILES |
[13CH3]Cl | |
Source | PubChem | |
URL | https://pubchem.ncbi.nlm.nih.gov | |
Description | Data deposited in or computed by PubChem | |
Molecular Formula |
CH3Cl | |
Source | PubChem | |
URL | https://pubchem.ncbi.nlm.nih.gov | |
Description | Data deposited in or computed by PubChem | |
DSSTOX Substance ID |
DTXSID20583926 | |
Record name | Chloro(~13~C)methane | |
Source | EPA DSSTox | |
URL | https://comptox.epa.gov/dashboard/DTXSID20583926 | |
Description | DSSTox provides a high quality public chemistry resource for supporting improved predictive toxicology. | |
Molecular Weight |
51.48 g/mol | |
Source | PubChem | |
URL | https://pubchem.ncbi.nlm.nih.gov | |
Description | Data deposited in or computed by PubChem | |
CAS No. |
19961-13-8 | |
Record name | Chloro(~13~C)methane | |
Source | EPA DSSTox | |
URL | https://comptox.epa.gov/dashboard/DTXSID20583926 | |
Description | DSSTox provides a high quality public chemistry resource for supporting improved predictive toxicology. | |
Record name | 19961-13-8 | |
Source | European Chemicals Agency (ECHA) | |
URL | https://echa.europa.eu/information-on-chemicals | |
Description | The European Chemicals Agency (ECHA) is an agency of the European Union which is the driving force among regulatory authorities in implementing the EU's groundbreaking chemicals legislation for the benefit of human health and the environment as well as for innovation and competitiveness. | |
Explanation | Use of the information, documents and data from the ECHA website is subject to the terms and conditions of this Legal Notice, and subject to other binding limitations provided for under applicable law, the information, documents and data made available on the ECHA website may be reproduced, distributed and/or used, totally or in part, for non-commercial purposes provided that ECHA is acknowledged as the source: "Source: European Chemicals Agency, http://echa.europa.eu/". Such acknowledgement must be included in each copy of the material. ECHA permits and encourages organisations and individuals to create links to the ECHA website under the following cumulative conditions: Links can only be made to webpages that provide a link to the Legal Notice page. | |
Retrosynthesis Analysis
AI-Powered Synthesis Planning: Our tool employs the Template_relevance Pistachio, Template_relevance Bkms_metabolic, Template_relevance Pistachio_ringbreaker, Template_relevance Reaxys, Template_relevance Reaxys_biocatalysis model, leveraging a vast database of chemical reactions to predict feasible synthetic routes.
One-Step Synthesis Focus: Specifically designed for one-step synthesis, it provides concise and direct routes for your target compounds, streamlining the synthesis process.
Accurate Predictions: Utilizing the extensive PISTACHIO, BKMS_METABOLIC, PISTACHIO_RINGBREAKER, REAXYS, REAXYS_BIOCATALYSIS database, our tool offers high-accuracy predictions, reflecting the latest in chemical research and data.
Strategy Settings
Precursor scoring | Relevance Heuristic |
---|---|
Min. plausibility | 0.01 |
Model | Template_relevance |
Template Set | Pistachio/Bkms_metabolic/Pistachio_ringbreaker/Reaxys/Reaxys_biocatalysis |
Top-N result to add to graph | 6 |
Feasible Synthetic Routes
Avertissement et informations sur les produits de recherche in vitro
Veuillez noter que tous les articles et informations sur les produits présentés sur BenchChem sont destinés uniquement à des fins informatives. Les produits disponibles à l'achat sur BenchChem sont spécifiquement conçus pour des études in vitro, qui sont réalisées en dehors des organismes vivants. Les études in vitro, dérivées du terme latin "in verre", impliquent des expériences réalisées dans des environnements de laboratoire contrôlés à l'aide de cellules ou de tissus. Il est important de noter que ces produits ne sont pas classés comme médicaments et n'ont pas reçu l'approbation de la FDA pour la prévention, le traitement ou la guérison de toute condition médicale, affection ou maladie. Nous devons souligner que toute forme d'introduction corporelle de ces produits chez les humains ou les animaux est strictement interdite par la loi. Il est essentiel de respecter ces directives pour assurer la conformité aux normes légales et éthiques en matière de recherche et d'expérimentation.