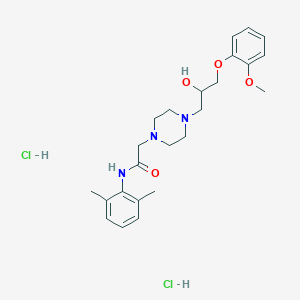
Ranolazine dihydrochloride
Vue d'ensemble
Description
Ranolazine dihydrochloride (chemical name: N-(2,6-dimethylphenyl)-4(2-hydroxy-3-[2-methoxyphenoxyl]propyl)-1-piperazineacetamide dihydrochloride) is a late sodium current inhibitor approved by the U.S. FDA in 2006 for chronic angina management in patients unresponsive to first-line therapies . It exerts anti-ischemic effects without significantly affecting heart rate or blood pressure, distinguishing it from traditional antianginal agents like β-blockers or calcium channel blockers. Its mechanism involves dual inhibition of cardiac late inward sodium current (INa) and rapid delayed rectifier potassium current (IKr), with reported IC50 values of 6 μM and 12 μM, respectively . Additionally, it partially inhibits fatty acid oxidation, shifting myocardial metabolism toward glucose utilization .
Ranolazine is administered as a sustained-release tablet with a half-life of ~7 hours, achieving steady-state concentrations within 3 days of twice-daily dosing. It is metabolized primarily by CYP3A4 and excreted renally, necessitating dose adjustments in patients with hepatic or moderate-to-severe renal impairment .
Méthodes De Préparation
Voies de synthèse et conditions réactionnelles
La synthèse du dihydrochlorure de ranolazine implique plusieurs étapes clés. Une méthode courante commence par la réaction du 2-méthoxyphénol avec l'épichlorhydrine en présence d'eau, de dioxane et d'hydroxyde de sodium pour former le 1-(2-méthoxyphénoxy)-2,3-époxypropane . Cet intermédiaire est ensuite condensé avec la pipérazine dans l'éthanol pour produire le 2-(2-méthoxyphénoxy)-1-(pipérazin-1-yl)éthanol . La dernière étape consiste à faire réagir ce composé avec la 2,6-diméthylaniline et le chlorure de chloroacétyle en présence de triéthylamine et de dichlorométhane pour produire la ranolazine .
Méthodes de production industrielle
La production industrielle du dihydrochlorure de ranolazine suit généralement des voies de synthèse similaires, mais à plus grande échelle. Le processus implique un contrôle minutieux des conditions réactionnelles pour garantir un rendement élevé et une pureté optimale. Des techniques de pointe telles que la chromatographie liquide haute performance (CLHP) sont utilisées pour surveiller la synthèse et garantir la qualité du produit final .
Analyse Des Réactions Chimiques
Types de réactions
Le dihydrochlorure de ranolazine subit diverses réactions chimiques, notamment :
Oxydation : La ranolazine peut être oxydée dans des conditions spécifiques, conduisant à la formation de divers produits d'oxydation.
Réduction : Le composé peut également subir des réactions de réduction, bien que celles-ci soient moins fréquentes.
Substitution : La ranolazine peut participer à des réactions de substitution, en particulier celles impliquant ses groupes fonctionnels.
Réactifs et conditions courants
Oxydation : Les agents oxydants courants comprennent le permanganate de potassium et le peroxyde d'hydrogène.
Réduction : Des agents réducteurs tels que l'hydrure de lithium aluminium peuvent être utilisés.
Substitution : Les conditions des réactions de substitution varient en fonction des groupes fonctionnels spécifiques impliqués.
Principaux produits
Les principaux produits formés à partir de ces réactions dépendent des conditions et des réactifs spécifiques utilisés. Par exemple, l'oxydation peut conduire à la formation de dérivés hydroxylés, tandis que les réactions de substitution peuvent produire divers dérivés de la pipérazine substitués .
Applications de la recherche scientifique
Le dihydrochlorure de ranolazine a un large éventail d'applications en recherche scientifique :
Biologie : La recherche a exploré ses effets sur le métabolisme cellulaire et le fonctionnement des canaux ioniques.
Mécanisme d'action
Le dihydrochlorure de ranolazine exerce ses effets principalement en inhibant la phase tardive du courant sodique entrant (INa) pendant la repolarisation cardiaque . Cette inhibition réduit les niveaux de sodium intracellulaire, ce qui à son tour diminue la surcharge en calcium dans les cellules cardiaques. La réduction de la surcharge en calcium contribue à améliorer la relaxation myocardique et à réduire la tension pariétale diastolique, ce qui soulage les symptômes de l'angine de poitrine . De plus, il a été démontré que la ranolazine inhibe d'autres canaux ioniques, notamment les canaux potassiques, ce qui peut contribuer à ses effets antiarythmiques .
Applications De Recherche Scientifique
Pharmacological Mechanism
Ranolazine functions by inhibiting the late sodium current (I_Na) in cardiac myocytes, which helps to reduce intracellular calcium overload. This mechanism lowers myocardial oxygen consumption and wall tension, making it effective in treating ischemic conditions. Additionally, ranolazine exhibits partial fatty acid oxidation inhibition, promoting glucose oxidation in ischemic myocytes and enhancing cardiac efficiency during stress conditions .
Chronic Stable Angina
Ranolazine is primarily indicated for chronic stable angina, often used as a second-line treatment when conventional therapies are insufficient. Clinical trials have demonstrated its efficacy in improving exercise tolerance and reducing angina frequency without significantly affecting heart rate or blood pressure .
Atrial Fibrillation
Emerging evidence suggests that ranolazine may be beneficial in managing atrial fibrillation (AF), particularly post-cardioversion. It has shown promise in increasing atrial refractory periods and reducing the incidence of AF in patients undergoing coronary artery bypass grafting (CABG) .
Heart Failure and Diastolic Dysfunction
Ranolazine has been investigated for its potential benefits in heart failure with preserved ejection fraction (HFpEF) and diastolic dysfunction. By improving diastolic function and reducing end-diastolic pressure, it may provide symptomatic relief in these populations .
Pulmonary Hypertension
Recent studies have explored ranolazine's role in pulmonary arterial hypertension (PAH). Its vasodilatory effects and ability to modulate cardiac function make it a candidate for further investigation in this area .
Chemotherapy-Induced Cardiotoxicity
Ranolazine is being evaluated for its protective effects against cardiotoxicity associated with certain chemotherapeutic agents. Preliminary studies indicate it may mitigate damage to cardiac myocytes during chemotherapy .
Diabetes Management
Interestingly, ranolazine has shown anti-glycemic effects, leading to reductions in hemoglobin A1c levels among diabetic patients. This attribute expands its potential utility beyond cardiovascular conditions .
Research Findings and Case Studies
Recent literature highlights several key studies demonstrating the efficacy of ranolazine across various conditions:
Mécanisme D'action
Ranolazine dihydrochloride exerts its effects primarily by inhibiting the late phase of the inward sodium current (INa) during cardiac repolarization . This inhibition reduces intracellular sodium levels, which in turn decreases calcium overload in cardiac cells. The reduction in calcium overload helps to improve myocardial relaxation and reduce diastolic wall tension, thereby alleviating symptoms of angina . Additionally, ranolazine has been shown to inhibit other ion channels, including potassium channels, which may contribute to its antiarrhythmic effects .
Comparaison Avec Des Composés Similaires
Pharmacological Targets and Mechanisms
The table below compares ranolazine dihydrochloride with structurally or functionally related compounds:
Compound | Primary Targets | IC50/EC50 | Mechanism | Indications |
---|---|---|---|---|
This compound | Late INa, IKr | INa: 6 μM; IKr: 12 μM | Late sodium current inhibition; partial fatty acid oxidation inhibition | Chronic angina, arrhythmia research |
Mexiletine HCl | Voltage-gated Na+ channels (Phase 0) | ~50–100 μM | Fast sodium channel blockade (Class IB antiarrhythmic) | Ventricular arrhythmias |
Flecainide acetate | Na+ channels (Phases 0 and 2) | ~1–3 μM | Prolonged sodium channel inactivation (Class IC antiarrhythmic) | Atrial fibrillation, supraventricular tachycardia |
Dofetilide | IKr (hERG channels) | IC50: 10–50 nM | Pure delayed rectifier potassium current blockade (Class III antiarrhythmic) | Atrial fibrillation/flutter |
GS-6615 (Eleclazine) | Late INa | ~0.3 μM | Selective late sodium current inhibition | Investigational for Long QT syndrome |
Ranolazine-d8 dihydrochloride | Same as ranolazine | Equivalent to ranolazine | Deuterated form for metabolic and pharmacokinetic studies | Research use only |
Key Insights :
- Unlike mexiletine and flecainide, which target peak sodium currents, ranolazine specifically inhibits late sodium currents, reducing intracellular calcium overload and arrhythmia susceptibility without depressing contractility .
- Dofetilide’s exclusive IKr blockade increases arrhythmia risk (e.g., torsades de pointes), whereas ranolazine’s dual INa/IKr action may confer a safer profile .
- GS-6615 (eleclazine) shares ranolazine’s late INa inhibition but lacks fatty acid oxidation effects, suggesting narrower therapeutic utility .
Clinical Efficacy and Indications
- Ranolazine: Reduces angina frequency and improves exercise tolerance in stable coronary artery disease . Off-label use in atrial fibrillation (AFib) suppression is supported by preclinical models showing reduced rotor complexity .
- Mexiletine/Flecainide : Effective for ventricular and supraventricular arrhythmias but contraindicated in structural heart disease due to proarrhythmic risks .
- Dofetilide : Approved for AFib/flutter but requires hospitalization for initiation due to QT prolongation risks .
Pharmacokinetic Profiles
Parameter | Ranolazine | Mexiletine | Flecainide | Dofetilide |
---|---|---|---|---|
Half-life (hrs) | 7 | 10–12 | 12–27 | 7–10 |
Metabolism | CYP3A4 > CYP2D6 | CYP2D6 | CYP2D6 | CYP3A4 |
Renal Excretion | ~5% unchanged | ~10% unchanged | ~30% unchanged | ~80% unchanged |
Drug Interactions | CYP3A4/P-gp inhibitors (e.g., verapamil) increase exposure | Limited | Avoid with β-blockers, amiodarone | Avoid QT-prolonging drugs |
Notable Differences:
- Ranolazine’s CYP3A4-dependent metabolism contrasts with mexiletine/flecainide’s reliance on CYP2D6, affecting co-administration with common cardiovascular drugs .
- Dofetilide’s renal excretion mandates strict dose adjustments in renal impairment, unlike ranolazine .
Structural and Functional Analogues
- Ranolazine Related Compound B: A monohydrate derivative with altered pharmacokinetics; used as a reference standard in analytical studies .
- Ranolazine-d8 dihydrochloride: Deuterated form for tracing drug metabolism without altering pharmacological activity .
Q & A
Basic Research Questions
Q. What are the primary molecular targets of ranolazine dihydrochloride, and how are they experimentally validated?
this compound primarily inhibits the late phase of the inward sodium current (INa) and the rapid delayed rectifier potassium current (IKr), with IC50 values of 6 µM and 12 µM, respectively . These targets are validated using electrophysiological assays (e.g., patch-clamp studies) and Langendorff-perfused heart models to assess ion channel activity and metabolic effects . Computational docking studies, such as Rosetta atomistic modeling, can further predict binding affinities to sodium and calcium channels .
Q. What experimental models are suitable for studying ranolazine's anti-ischemic effects?
- In vitro : Isolated working heart models (e.g., Langendorff preparations) under varying perfusion conditions (e.g., low calcium, high fatty acid) to measure glucose oxidation and ATP production .
- In vivo : Rodent models of induced ischemia-reperfusion injury to assess functional recovery and metabolic shifts .
- Cell-based : A549 cells treated pre- and post-viral infection to evaluate anti-influenza activity via EC50 calculations (e.g., 157.1 µg/ml vs. 208 µg/ml post-infection) .
Q. How should this compound be prepared and stored to ensure stability in laboratory settings?
- Stock solutions : Prepare in water or DMSO at 10 mM, aliquot to avoid freeze-thaw cycles, and store at -20°C for ≤2 years. Deuterated forms (e.g., Ranolazine-d8) require inert atmospheres to prevent isotopic exchange .
- Handling : Use protective equipment (gloves, goggles) and fume hoods, as degradation products may pose hazards .
Advanced Research Questions
Q. How can researchers resolve discrepancies in reported IC50 values for ranolazine's ion channel effects?
- Standardize assays : Use consistent cell lines (e.g., HEK293 expressing hERG channels for IKr) and buffer conditions (pH, temperature).
- Cross-validate methods : Compare patch-clamp data with fluorescence-based assays (e.g., FLIPR for calcium flux) .
- Meta-analysis : Pool data from studies using identical protocols, adjusting for variables like ranolazine batch purity (>98% recommended) .
Q. What computational strategies optimize ranolazine's binding affinity for novel targets (e.g., viral proteins)?
- Hotspot identification : Use Rosetta or Schrödinger Suite to map interaction sites on targets like SARS-CoV-2 spike protein .
- In silico evolution : Design focused libraries via phage display-inspired algorithms to enhance binding kinetics .
- Molecular dynamics (MD) : Simulate ranolazine-membrane transporter interactions (e.g., fatty acid oxidation enzymes) to predict off-target effects .
Q. How do experimental designs account for ranolazine's dual metabolic and ion channel modulation in cardiac studies?
- Dose stratification : Test sub-therapeutic (1–10 µM) vs. therapeutic (≥20 µM) concentrations to isolate metabolic (glucose oxidation) vs. electrophysiological effects .
- Temporal controls : In ischemia-reperfusion models, administer ranolazine during ischemia vs. reperfusion to clarify mechanism timing .
- Multi-omics integration : Pair transcriptomics (e.g., HIF-1α pathways) with metabolomics (e.g., lactate/ATP ratios) to map systemic responses .
Q. What methodologies address ranolazine's stability challenges in long-term cell culture studies?
- Degradation monitoring : Use HPLC-MS to quantify ranolazine and impurities (e.g., Related Compound B) over time .
- Matrix supplementation : Add antioxidants (e.g., ascorbate) to cell media to mitigate oxidative degradation .
- Cryopreservation : Store treated cells in liquid nitrogen with cryoprotectants (e.g., DMSO) for delayed analysis .
Q. Methodological Best Practices
- Contradiction analysis : For conflicting data (e.g., antidiabetic vs. cardiotoxic effects), use factorial experimental designs to isolate variables like dosage and model species .
- Safety protocols : Adhere to OSHA standards for carcinogen handling (e.g., PPE, locked storage) and dispose of waste via certified facilities .
- Resource validation : Source ranolazine from suppliers with ≥98% purity certificates and avoid non-validated vendors (e.g., benchchem.com ) .
Propriétés
Numéro CAS |
95635-56-6 |
---|---|
Formule moléculaire |
C24H34ClN3O4 |
Poids moléculaire |
464.0 g/mol |
Nom IUPAC |
N-(2,6-dimethylphenyl)-2-[4-[2-hydroxy-3-(2-methoxyphenoxy)propyl]piperazin-1-yl]acetamide;hydrochloride |
InChI |
InChI=1S/C24H33N3O4.ClH/c1-18-7-6-8-19(2)24(18)25-23(29)16-27-13-11-26(12-14-27)15-20(28)17-31-22-10-5-4-9-21(22)30-3;/h4-10,20,28H,11-17H2,1-3H3,(H,25,29);1H |
Clé InChI |
HIWSKCRHAOKBSL-UHFFFAOYSA-N |
SMILES |
CC1=C(C(=CC=C1)C)NC(=O)CN2CCN(CC2)CC(COC3=CC=CC=C3OC)O.Cl.Cl |
SMILES canonique |
CC1=C(C(=CC=C1)C)NC(=O)CN2CCN(CC2)CC(COC3=CC=CC=C3OC)O.Cl |
Apparence |
Powder |
Solubilité |
>75.1 [ug/mL] (The mean of the results at pH 7.4) |
Synonymes |
43285, RS Dihydrochloride, Ranolazine HCl, Ranolazine Hydrochloride, Ranolazine N-(2,6-dimethylphenyl)-4-(2-hydroxy-3-(2-methoxyphenoxy)propyl)-1-piperazineacetamide Ranexa ranolazine Ranolazine Dihydrochloride Ranolazine HCl Ranolazine Hydrochloride renolazine RS 43285 RS 43285 193 RS 43285-193 RS 43285193 RS-43285 RS43285 |
Origine du produit |
United States |
Retrosynthesis Analysis
AI-Powered Synthesis Planning: Our tool employs the Template_relevance Pistachio, Template_relevance Bkms_metabolic, Template_relevance Pistachio_ringbreaker, Template_relevance Reaxys, Template_relevance Reaxys_biocatalysis model, leveraging a vast database of chemical reactions to predict feasible synthetic routes.
One-Step Synthesis Focus: Specifically designed for one-step synthesis, it provides concise and direct routes for your target compounds, streamlining the synthesis process.
Accurate Predictions: Utilizing the extensive PISTACHIO, BKMS_METABOLIC, PISTACHIO_RINGBREAKER, REAXYS, REAXYS_BIOCATALYSIS database, our tool offers high-accuracy predictions, reflecting the latest in chemical research and data.
Strategy Settings
Precursor scoring | Relevance Heuristic |
---|---|
Min. plausibility | 0.01 |
Model | Template_relevance |
Template Set | Pistachio/Bkms_metabolic/Pistachio_ringbreaker/Reaxys/Reaxys_biocatalysis |
Top-N result to add to graph | 6 |
Feasible Synthetic Routes
Avertissement et informations sur les produits de recherche in vitro
Veuillez noter que tous les articles et informations sur les produits présentés sur BenchChem sont destinés uniquement à des fins informatives. Les produits disponibles à l'achat sur BenchChem sont spécifiquement conçus pour des études in vitro, qui sont réalisées en dehors des organismes vivants. Les études in vitro, dérivées du terme latin "in verre", impliquent des expériences réalisées dans des environnements de laboratoire contrôlés à l'aide de cellules ou de tissus. Il est important de noter que ces produits ne sont pas classés comme médicaments et n'ont pas reçu l'approbation de la FDA pour la prévention, le traitement ou la guérison de toute condition médicale, affection ou maladie. Nous devons souligner que toute forme d'introduction corporelle de ces produits chez les humains ou les animaux est strictement interdite par la loi. Il est essentiel de respecter ces directives pour assurer la conformité aux normes légales et éthiques en matière de recherche et d'expérimentation.