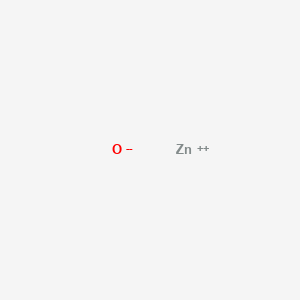
Zinc Oxide
Descripción general
Descripción
Zinc hydrate is a compound that consists of zinc ions (Zn²⁺) and water molecules. It is commonly found in various hydrated forms, such as zinc chloride hydrate (ZnCl₂·nH₂O) and zinc sulfate hydrate (ZnSO₄·nH₂O). These hydrates are colorless or white crystalline solids that are highly soluble in water . Zinc hydrate compounds are widely used in various industrial applications due to their unique chemical properties.
Métodos De Preparación
Synthetic Routes and Reaction Conditions
Zinc hydrate can be synthesized through various methods, including the reaction of zinc salts with water. For example, zinc chloride hydrate can be prepared by dissolving zinc chloride in water, resulting in the formation of different hydrates depending on the concentration and temperature . Another method involves the reaction of zinc sulfate with water to form zinc sulfate hydrate .
Industrial Production Methods
Industrial production of zinc hydrate often involves large-scale reactions of zinc salts with water. For instance, zinc sulfate hydrate is produced by dissolving zinc sulfate in water and allowing it to crystallize. The process can be controlled to obtain different hydrate forms by adjusting the temperature and concentration of the solution .
Análisis De Reacciones Químicas
Types of Reactions
Zinc hydrate undergoes various chemical reactions, including:
Oxidation: Zinc hydrate can be oxidized to form zinc oxide (ZnO) and water.
Reduction: Zinc hydrate can be reduced to elemental zinc and water.
Substitution: Zinc hydrate can undergo substitution reactions with other metal ions to form different metal hydrates.
Common Reagents and Conditions
Common reagents used in reactions with zinc hydrate include acids, bases, and other metal salts. For example, zinc hydrate reacts with sulfuric acid to form zinc sulfate and water . The reaction conditions, such as temperature and pH, play a crucial role in determining the products formed.
Major Products Formed
The major products formed from reactions involving zinc hydrate include this compound, zinc sulfate, and other metal hydrates. These products have various industrial applications, such as in the production of pigments, fertilizers, and pharmaceuticals .
Aplicaciones Científicas De Investigación
Zinc hydrate has numerous scientific research applications across various fields:
Mecanismo De Acción
The mechanism of action of zinc hydrate involves its interaction with various molecular targets and pathways. Zinc ions play a crucial role in enzyme function, protein synthesis, and cellular signaling . Zinc hydrate can also act as an antioxidant, protecting cells from oxidative stress by scavenging free radicals . Additionally, zinc hydrate can modulate the immune response by influencing the activity of immune cells .
Comparación Con Compuestos Similares
Zinc hydrate can be compared with other similar compounds, such as:
Zinc chloride hydrate: Similar to zinc hydrate, zinc chloride hydrate is highly soluble in water and used in various industrial applications.
Zinc acetate: Zinc acetate is another zinc compound that forms hydrates and is used in dietary supplements and pharmaceuticals.
Zinc borate hydrate: Zinc borate hydrate is used as a fire retardant and in the preservation of wood composites.
Zinc hydrate is unique due to its versatility and wide range of applications in different fields. Its ability to form various hydrates with distinct properties makes it a valuable compound in scientific research and industrial processes.
Propiedades
Fórmula molecular |
OZn |
---|---|
Peso molecular |
81.4 g/mol |
Nombre IUPAC |
zinc;oxygen(2-) |
InChI |
InChI=1S/O.Zn/q-2;+2 |
Clave InChI |
RNWHGQJWIACOKP-UHFFFAOYSA-N |
SMILES |
[O-2].[Zn+2] |
SMILES canónico |
[O-2].[Zn+2] |
Origen del producto |
United States |
Synthesis routes and methods I
Procedure details
Synthesis routes and methods II
Procedure details
Synthesis routes and methods III
Procedure details
Synthesis routes and methods IV
Procedure details
Synthesis routes and methods V
Procedure details
Descargo de responsabilidad e información sobre productos de investigación in vitro
Tenga en cuenta que todos los artículos e información de productos presentados en BenchChem están destinados únicamente con fines informativos. Los productos disponibles para la compra en BenchChem están diseñados específicamente para estudios in vitro, que se realizan fuera de organismos vivos. Los estudios in vitro, derivados del término latino "in vidrio", involucran experimentos realizados en entornos de laboratorio controlados utilizando células o tejidos. Es importante tener en cuenta que estos productos no se clasifican como medicamentos y no han recibido la aprobación de la FDA para la prevención, tratamiento o cura de ninguna condición médica, dolencia o enfermedad. Debemos enfatizar que cualquier forma de introducción corporal de estos productos en humanos o animales está estrictamente prohibida por ley. Es esencial adherirse a estas pautas para garantizar el cumplimiento de los estándares legales y éticos en la investigación y experimentación.