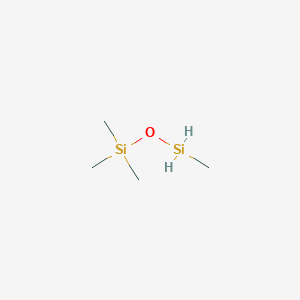
Tetramethyl disiloxane
Descripción general
Descripción
Tetramethyl disiloxane (CAS 3277-26-7), also known as 1,1,3,3-tetramethyldisiloxane, is a linear organosilicon compound with the molecular formula C₄H₁₄OSi₂ and a molecular weight of 134.32 g/mol . Its structure consists of two silicon atoms bonded to methyl groups and linked by an oxygen atom, forming a disiloxane backbone. Key physical properties include a boiling point of 70.1°C, a density of 0.75 g/cm³ at 20°C, and a refractive index of 1.3670 .
This compound is widely utilized as a precursor in silicone chemistry. For instance, it serves as a monomer in synthesizing poly(siloxane-urethane) copolymers, which exhibit enhanced phase miscibility, biocompatibility, and flame retardance . Additionally, it is employed in plasma-enhanced chemical vapor deposition (PECVD) for low-temperature glass coatings and as a reducing agent in organic synthesis .
Métodos De Preparación
Synthetic Routes and Reaction Conditions: Tetramethyl disiloxane is typically synthesized through the careful hydrolysis of chlorodimethylsilane. The reaction involves the addition of chlorodimethylsilane to water, which results in the formation of this compound and hydrochloric acid as a byproduct . The reaction must be carefully controlled to prevent the excessive generation of hydrochloric acid, which can be hazardous.
Industrial Production Methods: In industrial settings, this compound is produced using a similar hydrolysis process but on a larger scale. The reaction is carried out in a four-neck flask equipped with a stirring device, thermometer, rectifying tower, and condensing device. The reaction temperature is maintained between 10-20°C, and the reaction mixture is balanced for 30 minutes before heating for rapid rectification and extraction .
Análisis De Reacciones Químicas
Preparation of Tetramethyldisiloxane
The preparation of TMDS involves the reaction of organohydrogen polysiloxanes with methyl Grignard reagents, followed by hydrolysis. This process can be carried out in dialkyl ether solvents, and the hydrolysis step is typically performed at temperatures below 30°C using water or diluted aqueous solutions of hydrochloric acid, acetic acid, or alkali hydroxides .
Chemical Reactions Involving Tetramethyldisiloxane
TMDS is involved in a wide range of chemical reactions, including:
-
Amide Reductions : TMDS is effective in reducing amides to amines and aldehydes. This reaction is often catalyzed by transition metals such as platinum or iron complexes .
-
Nitro Group Reductions : TMDS can reduce nitro groups to amines, which is useful in synthesizing aromatic amines .
-
Nitrile Reductions : It is also capable of reducing nitriles to amines, providing a versatile route for amine synthesis .
-
Hydrogenolysis of Aryl-Chlorine Bonds : TMDS can facilitate the reduction of aryl-chlorine bonds, which is beneficial in the synthesis of complex organic molecules .
-
Reductive Formation of Sulfides : TMDS is used in the reductive formation of sulfides from sulfoxides or sulfones .
Table: Examples of Chemical Reactions Involving TMDS
Reaction Type | Substrate | Product | Catalyst/Conditions |
---|---|---|---|
Amide Reduction | Amides | Amines/Aldehydes | Pt, Fe complexes |
Nitro Reduction | Nitroarenes | Anilines | Various catalysts |
Nitrile Reduction | Nitriles | Amines | Transition metal catalysts |
Aryl-Chlorine Hydrogenolysis | Aryl Chlorides | Aryl Hydrocarbons | Pd, Pt catalysts |
Sulfide Formation | Sulfoxides/Sulfones | Sulfides | TMDS alone or with catalysts |
Mechanistic Insights and Selectivity
The reactivity of TMDS is often enhanced by the presence of two Si-H groups, which can participate in reactions more efficiently than monohydridic silanes. This "dual Si-H effect" allows for faster reaction rates and improved yields in certain transformations . The choice of catalysts can significantly influence the selectivity of TMDS-mediated reactions, enabling the reduction of specific functional groups while leaving others intact .
Comparison with Other Hydrosilanes
TMDS has been compared with other hydrosilanes such as phenylsilane and triethylsilane in various reactions. It generally offers superior results due to its higher reactivity and safety profile. For instance, TMDS is preferred over phenylsilane due to its lower risk of explosion and better tolerance for sensitive functional groups .
Industrial and Environmental Considerations
Aplicaciones Científicas De Investigación
Organic Synthesis
Reducing Agent
TMDSO is widely recognized for its role as a reducing agent in organic synthesis. It has been effectively utilized in:
- Platinum-Catalyzed Reductions : TMDSO facilitates the reduction of carboxamides to amines, showcasing its effectiveness under mild conditions and low catalyst loading .
- Hydrosilylation Reactions : The compound serves as a highly reactive reagent in the Au/TiO2-catalyzed hydrosilylation of carbonyl compounds, allowing for the reduction of aldehydes and ketones .
Case Study: Gold Nanoparticle Catalysis
Research by Ioannis N. et al. demonstrated that gold nanoparticles supported on TiO2 can catalyze the oxidative cycloaddition of TMDSO to alkynes at room temperature, yielding high product yields (up to 99%) while evolving hydrogen gas . This reaction highlights TMDSO's utility in producing complex organosilicon compounds.
Polymer Chemistry
Silicone Intermediate
TMDSO acts as a crucial intermediate in synthesizing various organopolysiloxanes and silicone polymers. Its applications include:
- End-Capping Agent : It is commonly used to modify the end groups of silicone polymers, enhancing their properties for specific applications .
- Hydrosilylation Reactions : TMDSO can react with vinyl-substituted silanes through hydrosilylation, leading to the formation of functionalized siloxanes which are vital in producing silicone elastomers and resins .
Materials Science
Atomic Layer Deposition (ALD) Precursor
In materials science, TMDSO is employed as a precursor in atomic layer deposition processes. Its ability to form thin films with precise thickness control makes it valuable for creating coatings and films with specific properties . The self-limiting nature of ALD allows for uniform deposition on complex surfaces.
Pharmaceutical Applications
TMDSO serves as an intermediate in synthesizing pharmaceutical compounds. Its reactivity allows for the incorporation of silicon into drug molecules, potentially enhancing their pharmacological properties and bioavailability .
Textile and Paper Treatments
The compound is also used as a waterproofing agent for textiles, paper, and leather products. This application leverages its hydrophobic properties to enhance water resistance and durability .
Summary Table of Applications
Application Area | Specific Uses | Key Benefits |
---|---|---|
Organic Synthesis | Reducing agent for carboxamides; hydrosilylation | Mild reaction conditions; high yields |
Polymer Chemistry | Silicone intermediate; end-capping agent | Tailored properties for silicone products |
Materials Science | ALD precursor | Precise film thickness; uniform deposition |
Pharmaceuticals | Intermediate in drug synthesis | Enhanced pharmacological properties |
Textiles & Paper | Waterproofing agent | Improved water resistance |
Mecanismo De Acción
The mechanism of action of tetramethyl disiloxane involves the transfer of hydride ions to the target molecule. This hydride transfer is facilitated by the presence of reactive Si-H groups in the molecular structure. The hydride ions reduce the target functional groups, resulting in the formation of the desired products . The reaction is often catalyzed by transition metals, which enhance the efficiency and selectivity of the hydride transfer .
Comparación Con Compuestos Similares
Comparison with Similar Compounds
The following table compares tetramethyl disiloxane with structurally related siloxanes, emphasizing molecular characteristics, reactivity, and applications:
Reactivity and Selectivity
- Hydrosilylation Performance : In reactions with allylbenzene, this compound achieved 72% conversion with 100% β-adduct selectivity , outperformed by cyclic siloxanes like D4 (96% conversion) but offering superior selectivity .
- Synthesis Efficiency : Modified hydrolysis methods for this compound derivatives (e.g., 1,3-bis(hydroxymethyl)tetramethyldisiloxane) reduced production time and solvent waste, yielding cross-linked polymers with 35–75 MPa tensile strength .
Functional Group Impact
- Hydroxyalkyl/Hydroxyester Derivatives: Functionalization with polar groups (e.g., hydroxypropyl or hydroxyhexanoyl) improves miscibility in polyurethane blends, enabling applications in biocompatible medical coatings .
- Vinyl vs. Methoxy Groups : Vinyl-substituted derivatives (e.g., divinyltetramethyldisiloxane) enhance cross-linking efficiency in elastomers, whereas methoxy groups increase hydrolytic stability for coatings .
Q & A
Basic Research Questions
Q. What are the standard methods for synthesizing and characterizing tetramethyl disiloxane derivatives?
Synthesis typically involves hydrolysis of chlorosilane precursors under controlled conditions (e.g., acidic or basic catalysis). For example, bis(hydroxyalkyl)-functionalized tetramethyl disiloxanes are synthesized via hydrosilylation reactions using platinum catalysts, followed by hydrolysis . Characterization relies on nuclear magnetic resonance (NMR) spectroscopy (¹H, ¹³C, ²⁹Si) to confirm molecular structure, infrared (IR) spectroscopy for functional group analysis, and elemental analysis for purity verification . For reproducibility, experimental details (e.g., solvent ratios, reaction times) must be meticulously documented .
Q. How is this compound utilized in materials science research?
Its primary applications include:
- Polymer modification : As a chain extender in poly(siloxane-urethane) copolymers to enhance phase miscibility, thermal stability, and mechanical properties (e.g., tensile strength up to 75 MPa) .
- Surface functionalization : As a coupling agent in composites to improve polymer-inorganic filler compatibility .
- Cross-linking : In silicone-based elastomers for controlled cross-link density, enabling tunable hardness (e.g., ~90 ShA) .
Q. What analytical techniques are critical for assessing purity and structural integrity?
- Chromatography : HPLC or GC-MS to verify purity (>98% by GC) .
- Spectroscopy : ²⁹Si NMR to confirm silicon bonding environments, avoiding misassignment of substituents .
- Thermal analysis : Differential scanning calorimetry (DSC) to detect phase transitions and decomposition thresholds .
Advanced Research Questions
Q. How can researchers resolve contradictions in reported thermal stability data for this compound-containing polymers?
Discrepancies often arise from variations in cross-link density, functional group placement, or residual catalysts. To address this:
- Perform thermogravimetric analysis (TGA) under inert and oxidative atmospheres to isolate decomposition mechanisms .
- Compare controlled synthesis batches (e.g., using identical siloxane:urethane ratios) to isolate variable impacts .
- Use small-angle X-ray scattering (SAXS) to correlate thermal stability with nanostructural homogeneity .
Q. What strategies optimize the synthesis of hydroxy-functionalized tetramethyl disiloxanes for high-yield applications?
Key methodologies include:
- Catalyst selection : Platinum(IV) chloride for efficient hydrosilylation, reducing side reactions .
- Solvent optimization : Polar aprotic solvents (e.g., DMSO) enhance reaction rates and yields in nucleophilic substitutions (e.g., Example 11, 94% yield) .
- Purification protocols : Semi-preparative HPLC to isolate isomers, critical for biomedical applications requiring enantiomeric purity .
Q. How can this compound derivatives improve electrolyte stability in lithium-ion batteries?
Borate-functionalized derivatives (e.g., PMBPDS) form stable cathode-electrolyte interphase (CEI) layers, scavenging hydrofluoric acid (HF) and mitigating transition metal dissolution. Electrochemical impedance spectroscopy (EIS) and X-ray photoelectron spectroscopy (XPS) are used to validate CEI composition and cycling performance (e.g., 70.6% capacity retention after 200 cycles at 4.5 V) .
Q. What experimental designs address phase separation challenges in siloxane-urethane copolymers?
- Monomer pre-organization : Pre-mixing disiloxanes with polyether polyols (e.g., Lupranol-1301) improves phase miscibility .
- Kinetic studies : Real-time FT-IR monitors urethane-siloxane reaction rates to optimize curing conditions (e.g., 115°C for 2 hours) .
- Mechanical testing : Dynamic mechanical analysis (DMA) correlates cross-link density with elastomer resilience .
Q. Methodological Considerations
- Reproducibility : Adhere to journal guidelines (e.g., Beilstein Journal protocols) for detailed experimental reporting, including supplementary data for compound characterization beyond five examples .
- Data validation : Cross-reference NMR assignments with computational models (e.g., DFT calculations for ²⁹Si chemical shifts) to minimize structural misinterpretation .
- Safety protocols : Follow EPA TSCA regulations (e.g., §721.10586) for handling siloxanes, particularly regarding aquatic toxicity mitigation .
Propiedades
IUPAC Name |
trimethyl(methylsilyloxy)silane | |
---|---|---|
Details | Computed by LexiChem 2.6.6 (PubChem release 2019.06.18) | |
Source | PubChem | |
URL | https://pubchem.ncbi.nlm.nih.gov | |
Description | Data deposited in or computed by PubChem | |
InChI |
InChI=1S/C4H14OSi2/c1-6-5-7(2,3)4/h6H2,1-4H3 | |
Details | Computed by InChI 1.0.5 (PubChem release 2019.06.18) | |
Source | PubChem | |
URL | https://pubchem.ncbi.nlm.nih.gov | |
Description | Data deposited in or computed by PubChem | |
InChI Key |
UHUUYVZLXJHWDV-UHFFFAOYSA-N | |
Details | Computed by InChI 1.0.5 (PubChem release 2019.06.18) | |
Source | PubChem | |
URL | https://pubchem.ncbi.nlm.nih.gov | |
Description | Data deposited in or computed by PubChem | |
Canonical SMILES |
C[SiH2]O[Si](C)(C)C | |
Details | Computed by OEChem 2.1.5 (PubChem release 2019.06.18) | |
Source | PubChem | |
URL | https://pubchem.ncbi.nlm.nih.gov | |
Description | Data deposited in or computed by PubChem | |
Molecular Formula |
C4H14OSi2 | |
Details | Computed by PubChem 2.1 (PubChem release 2019.06.18) | |
Source | PubChem | |
URL | https://pubchem.ncbi.nlm.nih.gov | |
Description | Data deposited in or computed by PubChem | |
Molecular Weight |
134.32 g/mol | |
Details | Computed by PubChem 2.1 (PubChem release 2021.05.07) | |
Source | PubChem | |
URL | https://pubchem.ncbi.nlm.nih.gov | |
Description | Data deposited in or computed by PubChem | |
Retrosynthesis Analysis
AI-Powered Synthesis Planning: Our tool employs the Template_relevance Pistachio, Template_relevance Bkms_metabolic, Template_relevance Pistachio_ringbreaker, Template_relevance Reaxys, Template_relevance Reaxys_biocatalysis model, leveraging a vast database of chemical reactions to predict feasible synthetic routes.
One-Step Synthesis Focus: Specifically designed for one-step synthesis, it provides concise and direct routes for your target compounds, streamlining the synthesis process.
Accurate Predictions: Utilizing the extensive PISTACHIO, BKMS_METABOLIC, PISTACHIO_RINGBREAKER, REAXYS, REAXYS_BIOCATALYSIS database, our tool offers high-accuracy predictions, reflecting the latest in chemical research and data.
Strategy Settings
Precursor scoring | Relevance Heuristic |
---|---|
Min. plausibility | 0.01 |
Model | Template_relevance |
Template Set | Pistachio/Bkms_metabolic/Pistachio_ringbreaker/Reaxys/Reaxys_biocatalysis |
Top-N result to add to graph | 6 |
Feasible Synthetic Routes
Descargo de responsabilidad e información sobre productos de investigación in vitro
Tenga en cuenta que todos los artículos e información de productos presentados en BenchChem están destinados únicamente con fines informativos. Los productos disponibles para la compra en BenchChem están diseñados específicamente para estudios in vitro, que se realizan fuera de organismos vivos. Los estudios in vitro, derivados del término latino "in vidrio", involucran experimentos realizados en entornos de laboratorio controlados utilizando células o tejidos. Es importante tener en cuenta que estos productos no se clasifican como medicamentos y no han recibido la aprobación de la FDA para la prevención, tratamiento o cura de ninguna condición médica, dolencia o enfermedad. Debemos enfatizar que cualquier forma de introducción corporal de estos productos en humanos o animales está estrictamente prohibida por ley. Es esencial adherirse a estas pautas para garantizar el cumplimiento de los estándares legales y éticos en la investigación y experimentación.