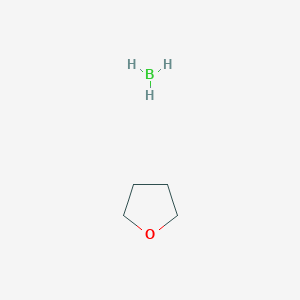
Borane;oxolane
Descripción general
Descripción
Borane;oxolane (IUPAC name: oxolane borane), commonly referred to as borane-tetrahydrofuran (BH₃·THF), is a Lewis acid-base complex formed by the coordination of borane (BH₃) with tetrahydrofuran (THF, oxolane). Its molecular formula is C₄H₁₁BO, with a molecular weight of 85.94 g/mol . This compound is widely used in organic synthesis for reductions, such as the hydrogenation of carbonyl groups and epoxide ring-opening reactions. For instance, it facilitates the reduction of nitroso intermediates to acetamides in carbohydrate chemistry . Its stability in THF solvent makes it a preferred reagent for controlled reductions under mild conditions .
Aplicaciones Científicas De Investigación
Organic Synthesis Applications
Borane-tetrahydrofuran is extensively used in organic synthesis due to its selective reducing properties. It can effectively reduce:
- Aldehydes and Ketones : Converts these functional groups into their corresponding alcohols.
- Carboxylic Acids and Esters : Reduces them to alcohols.
- Nitriles and Amides : Transforms them into amines.
The mechanism typically involves the formation of boron-oxygen bonds, which facilitate the transfer of hydride ions to the substrate. The efficiency of BTHF in these reductions makes it a preferred choice over other reducing agents.
Table 1: Reductive Capabilities of Borane-Tetrahydrofuran
Functional Group | Product Formed | Reaction Type |
---|---|---|
Aldehydes | Primary Alcohols | Reduction |
Ketones | Secondary Alcohols | Reduction |
Carboxylic Acids | Alcohols | Reduction |
Esters | Alcohols | Reduction |
Nitriles | Primary Amines | Reduction |
Amides | Primary Amines | Reduction |
Hydroboration Reactions
BTHF is widely utilized in hydroboration reactions, where it adds across carbon-carbon double bonds to form organoboranes. This process is crucial for synthesizing various organic compounds and intermediates.
Case Study: Hydroboration of Alkenes
In a study published by Purdue University, researchers demonstrated that BTHF could be used effectively for hydroborating alkenes to yield trialkylboranes. This method is advantageous due to the mild reaction conditions and high selectivity for the desired product .
Energy Storage and Hydrogen Production
Borane compounds, including BTHF, have been investigated for their potential in hydrogen storage systems. The ability of amine-boranes to release hydrogen gas upon thermal decomposition presents opportunities for clean energy applications.
Research Findings
A report from the Department of Energy highlighted that amine-boranes could meet the hydrogen storage targets set by the DOE by providing high weight percentages of hydrogen . The stability of these compounds under various conditions makes them suitable candidates for future hydrogen storage technologies.
Medical Applications
Recent studies have explored the use of borane complexes in medical settings, particularly as radioprotective agents. For example, phosphine-borane complexes have shown promise in protecting endothelial cells from radiation damage by modulating oxidative stress responses .
Table 2: Medical Applications of Borane Compounds
Application | Mechanism of Action | Reference |
---|---|---|
Radioprotection | Reduces oxidative stress post-irradiation | |
Drug Delivery | Facilitates targeted delivery mechanisms |
Safety Considerations
While borane-tetrahydrofuran is highly effective, safety concerns must be addressed due to its flammability and reactivity. Proper handling protocols are essential to mitigate risks associated with its use in laboratory settings .
Q & A
Q. Basic: What are the key methodological considerations for synthesizing compounds using borane-oxolane as a reducing agent?
Borane-oxolane (BH₃·THF) is widely used for selective reductions, such as converting nitriles to amines or reducing oximes. Key considerations include:
- Solvent Purity : Oxolane (tetrahydrofuran) must be anhydrous to prevent borane decomposition. Pre-dry with molecular sieves and monitor water content via Karl Fischer titration .
- Stoichiometry : Use a 1:1 molar ratio of borane to substrate for optimal yield, but adjust based on steric hindrance (e.g., bulky substrates may require excess borane) .
- Temperature Control : Reactions are typically conducted at 0–25°C; exothermic reactions require cooling to avoid side products.
- Work-Up : Quench excess borane with methanol or aqueous acid, followed by extraction to isolate products.
Q. Basic: How can researchers characterize borane-oxolane adducts and verify their stability in solution?
Characterization involves:
- NMR Spectroscopy : Monitor <sup>11</sup>B NMR for borane signals (δ ≈ 30–40 ppm for BH₃·THF). Shifts outside this range suggest decomposition or side reactions .
- FT-IR : Confirm B-H stretching vibrations at ~2100–2400 cm⁻¹.
- Stability Tests : Store solutions under inert gas (N₂/Ar) and track decomposition via periodic NMR or titration.
Q. Advanced: How can conformational dynamics of oxolane impact borane reactivity in reduction reactions?
Oxolane’s pseudorotational flexibility (e.g., envelope or twist conformers) influences borane coordination and reaction kinetics. For example:
- Solvent Effects : Flexible oxolane conformers stabilize borane-substrate intermediates, enhancing reduction rates. Use molecular dynamics (MD) simulations to model solvent conformer populations .
- NMR Coupling Constants : Measure <sup>3</sup>JHH values in oxolane to infer dominant conformers and correlate with reaction outcomes. Avoid oversimplified two-state models, as barrier-crossing conformers (e.g., 3T2/2T3) contribute significantly .
Q. Advanced: How should researchers resolve contradictions between computational predictions and experimental data in borane-oxolane-mediated reactions?
Discrepancies often arise from oversimplified computational models. Mitigate by:
- Multi-Scale Modeling : Combine quantum mechanics (QM) for reaction pathways with MD for solvent effects .
- Experimental Validation : Use in-situ <sup>11</sup>B NMR to track borane speciation during reactions. Compare with calculated thermodynamic parameters (e.g., Gibbs free energy barriers) .
- Systematic Reviews : Apply Cochrane methods to analyze literature data, identify bias (e.g., solvent purity variations), and reconcile conflicting results .
Q. Advanced: What strategies optimize borane-oxolane selectivity in stereochemically complex reductions?
To control stereoselectivity:
- Chiral Additives : Introduce chiral ligands (e.g., oxazaborolidines) to direct borane’s approach .
- Temperature Gradients : Lower temperatures favor kinetic control, stabilizing specific transition states.
- Substrate Engineering : Modify steric/electronic properties of substrates to bias borane coordination. Validate via X-ray crystallography of intermediates .
Q. Advanced: How can researchers design experiments to elucidate borane-oxolane’s role in multi-step synthetic pathways?
- Mechanistic Probes : Use isotopically labeled substrates (e.g., <sup>2</sup>H or <sup>13</sup>C) and track intermediates via LC-MS or NMR .
- Kinetic Isotope Effects (KIE) : Compare reaction rates with deuterated vs. non-deuterated substrates to identify rate-determining steps.
- Cross-Validation : Replicate published protocols with strict adherence to experimental details (e.g., inert atmosphere, reagent purity) to isolate variables causing reproducibility issues .
Q. Methodological: How should researchers document borane-oxolane experiments to ensure reproducibility?
Follow journal guidelines (e.g., Beilstein Journal of Organic Chemistry):
- Experimental Section : Specify borane concentration, solvent drying methods, and quenching protocols. For novel compounds, provide full characterization (NMR, HRMS, elemental analysis) .
- Supporting Information : Include raw NMR spectra, computational data, and failed attempts to highlight boundary conditions.
- Data Availability : Deposit datasets in repositories like Zenodo with metadata compliant with FAIR principles .
Q. Methodological: What systematic approaches address contradictory literature on borane-oxolane reaction mechanisms?
- Meta-Analysis : Use PRISMA guidelines to screen studies, extract data (e.g., yields, conditions), and apply statistical tests (ANOVA) to identify outliers .
- Sensitivity Analysis : Model how variables (e.g., temperature, solvent purity) affect outcomes using tools like R or Python.
- Collaborative Peer Review : Engage methodologists to critique experimental designs and suggest replication studies .
Comparación Con Compuestos Similares
Structural and Functional Analogues
Oxetane (C₃H₆O)
- Structure : A four-membered oxygen-containing heterocycle with significant ring strain due to its planar geometry .
- Reactivity : Oxetane’s ring strain enhances its utility in polymerizations and drug design. For example, it serves as a bioisostere for carbonyl groups in medicinal chemistry, improving metabolic stability .
- Applications : Key in synthesizing antiviral agents and self-healing polymers, where its low activation energy (~24 kcal/mol) enables reversible ring-opening .
Oxane (Tetrahydropyran, C₅H₁₀O)
- Structure : A six-membered oxygen heterocycle with a chair conformation, reducing ring strain compared to oxolane or oxetane .
- Reactivity : Less reactive due to minimal strain but used in glycosylation reactions and as a protecting group in natural product synthesis .
Boranephosphonate DNA Analogs
- Structure : Phosphorus(III) complexes with borane (e.g., compound 24a/b in ), where borane stabilizes the P(III) center .
- Reactivity : Mimic phosphate diesters in DNA backbones but with enhanced nuclease resistance and cellular uptake .
- Applications : Used in antisense oligonucleotides and gene therapy .
Reactivity and Thermodynamic Profiles
- Borane;oxolane : Acts as a reducing agent via BH₃ coordination, contrasting with oxetane/oxolane derivatives that undergo ring-opening or polymerization .
- Substituent Effects : Vinyl groups on oxolane (e.g., compound 24) lower activation energy by 8 kcal/mol compared to saturated analogs, enabling exergonic ring-opening .
Métodos De Preparación
Classical Synthesis via Sodium Borohydride and Boron Trifluoride
The most widely documented method involves the reaction of sodium borohydride (NaBH₄) with boron trifluoride diethyl etherate (BF₃·OEt₂) in THF solvent. This exothermic process generates BH₃-THF alongside sodium fluoride and hydrogen gas :
4 + 4 \, \text{BF}3 \rightarrow 3 \, \text{NaBF}4 + 2 \, \text{B}2\text{H}6 + 6 \, \text{H}2
The diborane (B₂H₆) intermediate reacts instantaneously with THF to form the adduct:
2\text{H}6 + 2 \, \text{THF} \rightarrow 2 \, \text{BH}_3\text{-THF}
Reaction Parameter Optimization
-
Stoichiometry : A 1:1.33 molar ratio of NaBH₄ to BF₃·OEt₂ maximizes yield (85–90%) while minimizing unreacted starting materials .
-
Temperature Control : Maintaining the reaction at −10°C to 0°C prevents THF oxidation and borane dimerization .
-
Solvent Purity : Anhydrous THF (<50 ppm water) is essential to avoid hydrolysis to boric acid .
Purification and Stabilization
Crude BH₃-THF solutions contain residual NaBF₄ and diborane. Filtration under inert atmosphere, followed by distillation at reduced pressure (40–60 mmHg, 30–40°C), yields a 1.0 M solution . Stabilizers like 0.005% tributylamine are added to inhibit THF peroxidation during storage .
Lewis Acid-Base Adduct Method (Patent CN104086576A)
This industrial-scale approach leverages Lewis acid-base interactions to produce high-purity BH₃ gas, subsequently complexed with THF .
Stepwise Procedure
-
Lewis Base Activation : Sodium borohydride (0.5–1.5 mol) is dissolved in THF under nitrogen.
-
Lewis Acid Addition : Gaseous BF₃ is introduced at −30°C to 50°C, generating BH₃ and NaBF₄:
-
Gas Purification : BH₃ is passed through a low-temperature cooling tower (−50°C) to remove BF₃, then absorbed into THF at 20°C.
Yield and Purity Data
Alternative Preparation Routes
Hydroboration of Alkenes
In-situ BH₃-THF formation occurs during hydroboration of alkenes like cyclohexene. The reaction proceeds via dicyclohexylborane intermediates, which dissolve in THF upon further alkene addition :
3\text{-THF} + 3 \, \text{C}6\text{H}{10} \rightarrow (\text{C}6\text{H}{11})3\text{B} + \text{THF}
This method is limited to laboratory-scale syntheses due to poor stoichiometric control .
Borane Dimethylsulfide (BMS) Transcomplexation
BH₃-THF can be prepared by displacing dimethyl sulfide (DMS) from borane-dimethylsulfide complexes:
3\text{-SMe}2 + \text{THF} \rightarrow \text{BH}3\text{-THF} + \text{SMe}2
This method avoids pyrophoric diborane but requires rigorous DMS removal via vacuum distillation .
Propiedades
IUPAC Name |
borane;oxolane | |
---|---|---|
Details | Computed by LexiChem 2.6.6 (PubChem release 2019.06.18) | |
Source | PubChem | |
URL | https://pubchem.ncbi.nlm.nih.gov | |
Description | Data deposited in or computed by PubChem | |
InChI |
InChI=1S/C4H8O.BH3/c1-2-4-5-3-1;/h1-4H2;1H3 | |
Details | Computed by InChI 1.0.5 (PubChem release 2019.06.18) | |
Source | PubChem | |
URL | https://pubchem.ncbi.nlm.nih.gov | |
Description | Data deposited in or computed by PubChem | |
InChI Key |
RMCYTHFAWCWRFA-UHFFFAOYSA-N | |
Details | Computed by InChI 1.0.5 (PubChem release 2019.06.18) | |
Source | PubChem | |
URL | https://pubchem.ncbi.nlm.nih.gov | |
Description | Data deposited in or computed by PubChem | |
Canonical SMILES |
B.C1CCOC1 | |
Details | Computed by OEChem 2.1.5 (PubChem release 2019.06.18) | |
Source | PubChem | |
URL | https://pubchem.ncbi.nlm.nih.gov | |
Description | Data deposited in or computed by PubChem | |
Molecular Formula |
C4H11BO | |
Details | Computed by PubChem 2.1 (PubChem release 2019.06.18) | |
Source | PubChem | |
URL | https://pubchem.ncbi.nlm.nih.gov | |
Description | Data deposited in or computed by PubChem | |
Molecular Weight |
85.94 g/mol | |
Details | Computed by PubChem 2.1 (PubChem release 2021.05.07) | |
Source | PubChem | |
URL | https://pubchem.ncbi.nlm.nih.gov | |
Description | Data deposited in or computed by PubChem | |
Synthesis routes and methods I
Procedure details
Synthesis routes and methods II
Procedure details
Retrosynthesis Analysis
AI-Powered Synthesis Planning: Our tool employs the Template_relevance Pistachio, Template_relevance Bkms_metabolic, Template_relevance Pistachio_ringbreaker, Template_relevance Reaxys, Template_relevance Reaxys_biocatalysis model, leveraging a vast database of chemical reactions to predict feasible synthetic routes.
One-Step Synthesis Focus: Specifically designed for one-step synthesis, it provides concise and direct routes for your target compounds, streamlining the synthesis process.
Accurate Predictions: Utilizing the extensive PISTACHIO, BKMS_METABOLIC, PISTACHIO_RINGBREAKER, REAXYS, REAXYS_BIOCATALYSIS database, our tool offers high-accuracy predictions, reflecting the latest in chemical research and data.
Strategy Settings
Precursor scoring | Relevance Heuristic |
---|---|
Min. plausibility | 0.01 |
Model | Template_relevance |
Template Set | Pistachio/Bkms_metabolic/Pistachio_ringbreaker/Reaxys/Reaxys_biocatalysis |
Top-N result to add to graph | 6 |
Feasible Synthetic Routes
Descargo de responsabilidad e información sobre productos de investigación in vitro
Tenga en cuenta que todos los artículos e información de productos presentados en BenchChem están destinados únicamente con fines informativos. Los productos disponibles para la compra en BenchChem están diseñados específicamente para estudios in vitro, que se realizan fuera de organismos vivos. Los estudios in vitro, derivados del término latino "in vidrio", involucran experimentos realizados en entornos de laboratorio controlados utilizando células o tejidos. Es importante tener en cuenta que estos productos no se clasifican como medicamentos y no han recibido la aprobación de la FDA para la prevención, tratamiento o cura de ninguna condición médica, dolencia o enfermedad. Debemos enfatizar que cualquier forma de introducción corporal de estos productos en humanos o animales está estrictamente prohibida por ley. Es esencial adherirse a estas pautas para garantizar el cumplimiento de los estándares legales y éticos en la investigación y experimentación.