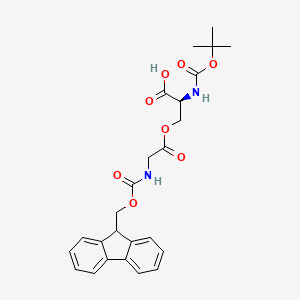
Boc-Ser(Fmoc-Gly)-OH
Descripción general
Descripción
Boc-Ser(Fmoc-Gly)-OH is a protected serine derivative featuring a tert-butoxycarbonyl (Boc) group on the α-amino group and a 9-fluorenylmethoxycarbonyl (Fmoc)-protected glycine moiety attached via an ester linkage to the serine hydroxyl group. This dual-protection strategy enables sequential deprotection in solid-phase peptide synthesis (SPPS), making it a critical building block for constructing complex peptides and proteins, such as collagen-like polymers and amyloid-β (Aβ1-42) .
Métodos De Preparación
Synthetic Routes for Boc-Ser(Fmoc-Gly)-OH
Sequential Protection Strategy
The synthesis begins with the orthogonal protection of serine’s α-amino and hydroxyl groups:
-
Boc Protection of Serine :
L-Serine is treated with di-tert-butyl dicarbonate (Boc₂O) in a basic aqueous solution (e.g., NaOH/THF/water) to yield Boc-Ser-OH. The Boc group ensures acid-labile protection of the α-amino group while leaving the hydroxyl group reactive for subsequent functionalization . -
Fmoc-Glycine Activation :
Fmoc-Gly-OH is activated using carbodiimide reagents such as DCC (N,N'-dicyclohexylcarbodiimide) or EDCI (1-ethyl-3-(3-dimethylaminopropyl)carbodiimide) in the presence of HOBt (hydroxybenzotriazole) or HOAt (1-hydroxy-7-azabenzotriazole). This step generates an active ester intermediate, facilitating nucleophilic attack by serine’s hydroxyl group . -
Esterification Reaction :
The activated Fmoc-Gly-OH is coupled to Boc-Ser-OH in anhydrous dichloromethane (DCM) or dimethylformamide (DMF) under inert atmosphere. Catalytic DMAP (4-dimethylaminopyridine) enhances reaction kinetics by deprotonating the serine hydroxyl group. The reaction typically proceeds at 0–25°C for 4–12 hours, yielding this compound .
Key Reaction Conditions:
Step | Reagents/Solvents | Temperature | Time |
---|---|---|---|
Boc Protection | Boc₂O, NaOH, THF/H₂O | 0–5°C | 2–4 hours |
Fmoc-Gly Activation | DCC/HOBt, DCM | 25°C | 1 hour |
Esterification | Boc-Ser-OH, DMAP, DCM/DMF | 0–25°C | 4–12 hours |
Solid-Phase Synthesis Adaptations
While this compound is typically prepared in solution phase, patent literature describes analogous strategies for resin-bound intermediates. For example, Fmoc-Gly-Wang resin is functionalized with Boc-Ser via ester linkages using HATU (1-[bis(dimethylamino)methylene]-1H-1,2,3-triazolo[4,5-b]pyridinium 3-oxid hexafluorophosphate) and DIEA (N,N-diisopropylethylamine) in DMF . This approach minimizes racemization and simplifies purification but requires acid-resistant resins to withstand Boc deprotection conditions .
Critical Parameters in Synthesis
Protecting Group Orthogonality
The Boc and Fmoc groups exhibit complementary stability:
-
Boc : Removed via trifluoroacetic acid (TFA) in DCM (25–50% v/v), preserving Fmoc and ester bonds .
-
Fmoc : Cleaved with piperidine (20% v/v in DMF), leaving Boc intact .
This orthogonality allows sequential deprotection during SPPS, enabling selective elongation of peptide chains .
Racemization Mitigation
Serine’s α-carbon is prone to racemization under basic conditions. To minimize this:
-
Reactions are conducted at low temperatures (0–5°C).
-
Coupling agents like HOAt or HATU are preferred over DCC due to their superior efficiency and reduced side reactions .
Purification and Characterization
Chromatographic Purification
Crude this compound is purified via reversed-phase high-performance liquid chromatography (RP-HPLC) using a C18 column and acetonitrile/water gradients. The product typically elutes at 60–70% acetonitrile, with a retention time of 12–15 minutes .
Analytical Validation
-
Mass Spectrometry : ESI-MS confirms the molecular ion peak at m/z 485.3 [M+H]⁺ .
-
Amino Acid Analysis : Hydrolysis with 6N HCl (110°C, 24 hours) yields serine and glycine in a 1:1 molar ratio .
Industrial-Scale Considerations
Patent WO2017114191A1 highlights scalability challenges and solutions:
-
Deprotection Efficiency : Pd(PPh₃)₄-mediated removal of Alloc groups (unrelated to Boc/Fmoc) demonstrates the utility of transition-metal catalysts in orthogonal protection schemes .
-
Solvent Selection : CH₂Cl₂ and DMF are preferred for large-scale reactions due to their low cost and compatibility with Boc/Fmoc chemistry .
Emerging Methodologies
Recent advances include:
Análisis De Reacciones Químicas
Types of Reactions: Boc-Ser(Fmoc-Gly)-OH can undergo various chemical reactions, including:
Deprotection Reactions: Removal of the Boc group using TFA and the Fmoc group using piperidine.
Coupling Reactions: Formation of peptide bonds using reagents like DIC and OxymaPure.
Substitution Reactions: Introduction of different functional groups at the amino or carboxyl termini.
Common Reagents and Conditions:
Deprotection: TFA for Boc removal, piperidine for Fmoc removal.
Coupling: DIC and OxymaPure in N-methyl-2-pyrrolidone (NMP) as the solvent.
Major Products: The primary product of these reactions is the desired peptide sequence with the protecting groups removed at the appropriate stages .
Aplicaciones Científicas De Investigación
Solid-Phase Peptide Synthesis
Overview of Fmoc and Boc Strategies
The Fmoc strategy has become the predominant method for peptide synthesis due to its compatibility with a range of modifications, including phosphorylated and glycosylated peptides. The Boc strategy, while less common now, remains relevant for specific applications where harsh deprotection conditions are not suitable. Boc-Ser(Fmoc-Gly)-OH serves as an important building block in these methods.
Benefits of Using this compound
- Low Epimerization Rates : The use of this compound minimizes epimerization during coupling reactions, which is critical for maintaining the integrity of peptide sequences. Studies show that Fmoc-based coupling can achieve epimerization levels as low as 0.18% at elevated temperatures .
- Stability and Compatibility : This compound exhibits excellent stability in solution and is compatible with various coupling reagents used in SPPS, making it a reliable choice for synthesizing complex peptides .
Glycosylation Applications
Glycosylated Peptide Synthesis
The incorporation of glycosylation into peptides enhances their biological activity and stability. This compound can be utilized to synthesize glycosylated peptide thioesters through the Boc strategy, which avoids the harsh conditions associated with Fmoc methods.
- Case Study : The synthesis of chitobiosylated peptide thioesters demonstrated that using this compound resulted in higher yields compared to Fmoc strategies. This was particularly evident in the synthesis of the extracellular matrix metalloproteinase inducer, emmprin .
Peptide Thioester Formation
Thioester Methodology
Thioesters are crucial intermediates in peptide synthesis, allowing for the formation of larger peptides through segment coupling. This compound has been employed effectively to create peptide thioesters that are essential for various biological applications.
- Experimental Findings : Research indicates that using Boc-protected serine derivatives like this compound leads to successful thioester formation without significant degradation of sensitive glycosidic linkages . This method has proven reliable for synthesizing complex peptides that require specific structural features.
Mecanismo De Acción
The mechanism of action of Boc-Ser(Fmoc-Gly)-OH is primarily related to its role in peptide synthesis. The Boc and Fmoc groups protect the amino acids during the synthesis process, preventing side reactions and ensuring the correct sequence of amino acids. The deprotection steps allow for the sequential addition of amino acids, ultimately leading to the formation of the desired peptide .
Comparación Con Compuestos Similares
Key Structural Features :
- Boc Group : Acid-labile, removed with trifluoroacetic acid (TFA).
- Fmoc-Gly Ester : Base-labile (cleaved with piperidine), allowing orthogonal deprotection .
- O-Acyl Isopeptide Unit : Facilitates sol–gel transitions in collagen-mimetic peptides via O–N acyl migration .
Below is a detailed comparison of Boc-Ser(Fmoc-Gly)-OH with structurally or functionally related compounds, focusing on synthesis, applications, and stability.
Table 1: Comparative Analysis of this compound and Analogues
Structural and Functional Differences
- Orthogonal Deprotection : this compound uniquely combines acid- and base-labile groups, enabling sequential assembly in SPPS. In contrast, Boc-Ser(TBDPS)-OH relies on the bulky TBDPS group for hydroxyl protection, which may hinder coupling efficiency .
- Hydroxyl Modifications : The Fmoc-Gly ester in this compound allows post-synthetic O–N acyl migration, critical for forming thermoresponsive gels in collagen-like peptides . Compounds like Boc-Ser(Me)-OH lack this dynamic functionality.
Stability and Handling
- Fmoc Sensitivity: this compound requires careful handling to prevent premature deprotection. Fmoc cleavage with 10% piperidine (vs. 20% for standard Fmoc-amino acids) minimizes side reactions .
- Solubility : Boc-Ser(Piv)-OH and Fmoc-L-Lys(Boc)-Gly-OH exhibit improved solubility in organic solvents compared to this compound, which may precipitate in DMF/CH₂Cl₂ mixtures .
Actividad Biológica
Boc-Ser(Fmoc-Gly)-OH is a derivative of serine that plays a significant role in peptide synthesis, particularly in the context of solid-phase peptide synthesis (SPPS). This compound is characterized by its unique structural features, including the Boc (tert-butyloxycarbonyl) protecting group on the amino group and the Fmoc (9-fluorenylmethoxycarbonyl) protecting group on the hydroxyl group. The biological activity of this compound can be explored through its role in peptide synthesis, stability, and potential applications in drug development.
Chemical Structure and Properties
The structure of this compound can be represented as follows:
Where:
- Boc : Protects the amino group.
- Fmoc : Protects the hydroxyl group.
- Gly : Represents glycine, which is attached to serine.
Table 1: Key Properties of this compound
Property | Value |
---|---|
Molecular Weight | 335.37 g/mol |
Solubility | Soluble in DMF, DMSO |
Melting Point | Not specified |
Stability | Stable under acidic conditions |
1. Role in Peptide Synthesis
This compound is primarily utilized in SPPS, where it serves as a building block for synthesizing peptides. The protection groups allow for selective reactions without interfering with other functional groups present in the peptide sequence. Its application has been shown to enhance yields and purities of peptides, particularly those prone to aggregation.
2. Isoacyl Dipeptides
The incorporation of this compound into isoacyl dipeptides has been noted for its ability to disrupt aggregation tendencies in peptides. This modification leads to improved solubility and facilitates purification processes during synthesis. Isoacyl dipeptides replace amide bonds with ester linkages, which significantly alters the conformation and stability of the resulting peptides .
3. Case Studies
Several studies have highlighted the effectiveness of this compound in various peptide synthesis scenarios:
- Study on Amyloidogenic Peptides : Research demonstrated that using isoacyl dipeptides containing this compound improved the solubility of amyloidogenic peptides, which are typically difficult to synthesize due to their propensity to aggregate .
- Fragment Condensation : The compound has been utilized effectively in fragment condensation reactions where its incorporation at the C-terminus allows for coupling without risk of epimerization, thus maintaining the integrity of sensitive sequences .
4. Stability and Reactivity
The stability of this compound under various conditions is crucial for its application in synthesis. It has shown resilience against racemization during coupling reactions, which is vital for maintaining enantiomeric purity in synthesized peptides. Research indicates that its use results in minimal side reactions when compared to traditional methods .
Discussion
The biological activity of this compound extends beyond mere structural utility; it plays a pivotal role in enhancing synthetic efficiency and improving the overall quality of peptide products. Its unique properties make it a valuable component in modern peptide synthesis strategies, particularly for complex sequences that require high fidelity and stability.
Q & A
Basic Research Questions
Q. What are the optimal deprotection conditions for Boc and Fmoc groups in Boc-Ser(Fmoc-Gly)-OH during solid-phase peptide synthesis?
- Methodological Answer :
- Boc Deprotection : Use trifluoroacetic acid (TFA) in dichloromethane (DCM) (1:1 v/v) for 30–60 minutes, followed by neutralization with 5% DIEA in DCM. This ensures selective removal of the Boc group without affecting the Fmoc-protected glycine .
- Fmoc Deprotection : Treat with 20% piperidine in DMF for 2 × 5 minutes to cleave the Fmoc group. Monitor completeness via UV absorbance at 301 nm .
- Key Considerations : Orthogonal protection allows sequential synthesis; confirm deprotection efficiency via HPLC or mass spectrometry .
Q. What analytical techniques are recommended for verifying the purity and stereochemical integrity of this compound post-synthesis?
- Methodological Answer :
- Purity Analysis : Use reverse-phase HPLC with a C18 column (gradient: 0.1% TFA in water/acetonitrile).
- Stereochemical Integrity : Employ and NMR to confirm retention of chiral centers. Optical rotation measurements ([α]) should align with literature values (e.g., [α] +20° for Boc-Ser derivatives) .
- Mass Confirmation : High-resolution mass spectrometry (HRMS) to verify molecular ion peaks (e.g., CHNO requires m/z 480.1762) .
Q. How does the orthogonal protection strategy using Boc and Fmoc groups facilitate the stepwise assembly of glycopeptides?
- Methodological Answer :
- Boc Stability : Resists basic conditions (e.g., piperidine), enabling selective Fmoc removal during chain elongation.
- Sequential Synthesis : Example workflow: (1) Deprotect Fmoc-Gly with piperidine, (2) couple next amino acid, (3) repeat. Final Boc removal occurs post-resin cleavage with HF or TFA .
- Advantage : Prevents premature deprotection, critical for synthesizing peptides with multiple post-translational modifications.
Advanced Research Questions
Q. How can researchers mitigate racemization risks during the coupling of this compound in automated peptide synthesizers?
- Methodological Answer :
- Coupling Agents : Use HOBt/DIC or Oxyma Pure/DIEA to minimize racemization. Avoid carbodiimides alone, which increase basicity and racemization risk .
- Temperature Control : Perform couplings at 0–4°C to slow base-catalyzed racemization.
- Monitoring : Analyze diastereomer formation via chiral HPLC post-cleavage. Racemization >1% warrants protocol revision .
Q. What strategies are effective in resolving solubility issues of this compound in non-polar solvents during solution-phase synthesis?
- Methodological Answer :
- Solvent Systems : Use DMF:DCM (1:4) or DMSO to enhance solubility. For highly hydrophobic sequences, add 0.1% TFA to protonate the amino group .
- Microwave-Assisted Synthesis : Increases solubility via localized heating (50–60°C) without degrading acid-labile groups .
- Validation : Confirm solubility via dynamic light scattering (DLS) or visual turbidity assays.
Q. In conformational studies, how does the incorporation of this compound influence peptide backbone flexibility compared to unprotected serine residues?
- Methodological Answer :
- Circular Dichroism (CD) : Compare α-helix or β-sheet content in protected vs. unprotected peptides. Boc/Fmoc groups may restrict rotation at the serine side chain, reducing conformational entropy .
- Molecular Dynamics (MD) Simulations : Analyze Ramachandran plots for serine φ/ψ angles. Protected serine often shows reduced flexibility in glycine-rich regions .
- Application : Critical for designing constrained peptides with enhanced receptor-binding specificity.
Propiedades
IUPAC Name |
(2S)-3-[2-(9H-fluoren-9-ylmethoxycarbonylamino)acetyl]oxy-2-[(2-methylpropan-2-yl)oxycarbonylamino]propanoic acid | |
---|---|---|
Source | PubChem | |
URL | https://pubchem.ncbi.nlm.nih.gov | |
Description | Data deposited in or computed by PubChem | |
InChI |
InChI=1S/C25H28N2O8/c1-25(2,3)35-24(32)27-20(22(29)30)14-33-21(28)12-26-23(31)34-13-19-17-10-6-4-8-15(17)16-9-5-7-11-18(16)19/h4-11,19-20H,12-14H2,1-3H3,(H,26,31)(H,27,32)(H,29,30)/t20-/m0/s1 | |
Source | PubChem | |
URL | https://pubchem.ncbi.nlm.nih.gov | |
Description | Data deposited in or computed by PubChem | |
InChI Key |
WYWURCSQLSKGCY-FQEVSTJZSA-N | |
Source | PubChem | |
URL | https://pubchem.ncbi.nlm.nih.gov | |
Description | Data deposited in or computed by PubChem | |
Canonical SMILES |
CC(C)(C)OC(=O)NC(COC(=O)CNC(=O)OCC1C2=CC=CC=C2C3=CC=CC=C13)C(=O)O | |
Source | PubChem | |
URL | https://pubchem.ncbi.nlm.nih.gov | |
Description | Data deposited in or computed by PubChem | |
Isomeric SMILES |
CC(C)(C)OC(=O)N[C@@H](COC(=O)CNC(=O)OCC1C2=CC=CC=C2C3=CC=CC=C13)C(=O)O | |
Source | PubChem | |
URL | https://pubchem.ncbi.nlm.nih.gov | |
Description | Data deposited in or computed by PubChem | |
Molecular Formula |
C25H28N2O8 | |
Source | PubChem | |
URL | https://pubchem.ncbi.nlm.nih.gov | |
Description | Data deposited in or computed by PubChem | |
Molecular Weight |
484.5 g/mol | |
Source | PubChem | |
URL | https://pubchem.ncbi.nlm.nih.gov | |
Description | Data deposited in or computed by PubChem | |
Descargo de responsabilidad e información sobre productos de investigación in vitro
Tenga en cuenta que todos los artículos e información de productos presentados en BenchChem están destinados únicamente con fines informativos. Los productos disponibles para la compra en BenchChem están diseñados específicamente para estudios in vitro, que se realizan fuera de organismos vivos. Los estudios in vitro, derivados del término latino "in vidrio", involucran experimentos realizados en entornos de laboratorio controlados utilizando células o tejidos. Es importante tener en cuenta que estos productos no se clasifican como medicamentos y no han recibido la aprobación de la FDA para la prevención, tratamiento o cura de ninguna condición médica, dolencia o enfermedad. Debemos enfatizar que cualquier forma de introducción corporal de estos productos en humanos o animales está estrictamente prohibida por ley. Es esencial adherirse a estas pautas para garantizar el cumplimiento de los estándares legales y éticos en la investigación y experimentación.