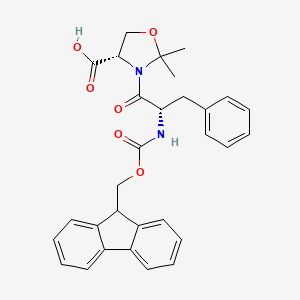
Fmoc-Phe-Ser(Psi(Me,Me)pro)-OH
Descripción general
Descripción
Fmoc-Phe-Ser(Psi(Me,Me)pro)-OH (CAS: 878797-01-4) is a pseudoproline-containing dipeptide derivative widely utilized in peptide synthesis and materials science. The compound features a fluorenylmethyloxycarbonyl (Fmoc) protecting group on the phenylalanine (Phe) residue and a pseudoproline [Ser(ΨMe,MePro)] moiety on the serine (Ser) residue. This structural modification enhances peptide solubility during solid-phase synthesis and disrupts secondary structures, facilitating challenging cyclization reactions such as ring-closing metathesis (RCM) . Its applications span drug discovery, nanotechnology (e.g., templating gold nanoparticles), and biochemical research .
Métodos De Preparación
Synthetic Routes and Reaction Conditions
The synthesis of Fmoc-Phe-Ser(Psi(Me,Me)pro)-OH typically involves the following steps:
Fmoc Protection: The amino group of phenylalanine is protected using the Fmoc group.
Coupling Reaction: The protected phenylalanine is coupled with serine, which is modified to include the pseudo-proline moiety.
Deprotection: The Fmoc group is removed under basic conditions to yield the final compound.
The reaction conditions often involve the use of coupling reagents such as N,N’-diisopropylcarbodiimide (DIC) and hydroxybenzotriazole (HOBt) to facilitate the formation of peptide bonds. Solvents like dimethylformamide (DMF) are commonly used to dissolve the reactants and reagents.
Industrial Production Methods
Industrial production of this compound follows similar synthetic routes but on a larger scale. Automated peptide synthesizers are often employed to ensure high yield and purity. The process involves:
Solid-Phase Peptide Synthesis (SPPS): This method allows for the sequential addition of amino acids to a growing peptide chain anchored to a solid resin.
Purification: High-performance liquid chromatography (HPLC) is used to purify the final product.
Análisis De Reacciones Químicas
Types of Reactions
Fmoc-Phe-Ser(Psi(Me,Me)pro)-OH undergoes various chemical reactions, including:
Oxidation: The serine residue can be oxidized to form a hydroxyl group.
Reduction: The pseudo-proline moiety can be reduced under specific conditions.
Substitution: The Fmoc group can be substituted with other protecting groups or functional groups.
Common Reagents and Conditions
Oxidation: Reagents like hydrogen peroxide (H2O2) or sodium periodate (NaIO4) are used.
Reduction: Reducing agents such as sodium borohydride (NaBH4) or lithium aluminum hydride (LiAlH4) are employed.
Substitution: Substitution reactions often involve nucleophiles like amines or thiols.
Major Products
Oxidation: Formation of hydroxylated derivatives.
Reduction: Formation of reduced pseudo-proline derivatives.
Substitution: Formation of substituted peptide derivatives with various functional groups.
Aplicaciones Científicas De Investigación
Peptide Synthesis
Overview : Fmoc-Phe-Ser(Psi(Me,Me)pro)-OH is widely recognized as a crucial building block in peptide synthesis. Its unique structure allows for the formation of complex peptide sequences with enhanced solubility and reduced aggregation during synthesis.
Key Benefits :
- Disruption of β-sheet Structures : The incorporation of pseudoproline units helps to disrupt β-sheet structures that often lead to intermolecular aggregation during chain elongation. This results in improved solvation and coupling kinetics .
- Facilitated Cyclization : The presence of pseudoproline enhances the cyclization of peptides, which is essential for creating cyclic peptides that exhibit improved stability and biological activity .
Drug Development
Overview : This compound is instrumental in the development of peptide-based therapeutics. Its ability to target specific biological pathways makes it a valuable asset in pharmaceutical research.
Applications :
- Therapeutic Peptides : this compound is utilized to design drugs that can effectively interact with biological targets, enhancing their therapeutic efficacy .
- Bioactivity Enhancement : The unique structural features of this compound can improve the bioactivity of therapeutic peptides, making them more effective in clinical settings .
Bioconjugation
Overview : Bioconjugation refers to the process of attaching biomolecules to peptides or proteins to enhance their functionality. This compound plays a vital role in this area.
Key Applications :
- Targeted Delivery Systems : By linking this dipeptide to other biomolecules, researchers can create targeted delivery systems for drug formulations, improving the specificity and efficacy of therapeutic agents .
- Diagnostic Tools : The compound is also explored for use in diagnostic applications where high specificity is required to detect biomarkers in clinical assays .
Research in Protein Engineering
Overview : this compound aids researchers in modifying proteins to study their structure and function.
Significance :
- Insights into Protein Structure : This compound allows for modifications that provide valuable insights into protein interactions and structure-function relationships, which are critical for understanding diseases and developing new treatments .
- Development of Novel Therapeutics : By enhancing the stability and activity of proteins, it contributes to the development of novel protein therapeutics that can be used in various medical applications .
Diagnostic Applications
Overview : The compound has potential applications in developing diagnostic tools that require high specificity.
Case Studies :
- Biomarker Detection Assays : this compound is being investigated for its role in creating assays that can accurately detect specific biomarkers related to diseases .
- Clinical Relevance : The ability to create highly specific assays using this compound may lead to advancements in clinical diagnostics and patient management strategies .
Data Table: Summary of Applications
Application Area | Key Features | Benefits |
---|---|---|
Peptide Synthesis | Disruption of β-sheet structures | Improved solvation and coupling kinetics |
Drug Development | Targeting specific biological pathways | Enhanced therapeutic efficacy |
Bioconjugation | Linking peptides to biomolecules | Creation of targeted delivery systems |
Protein Engineering | Modification for structural insights | Development of novel therapeutics |
Diagnostic Applications | High specificity assays | Accurate detection of biomarkers |
Mecanismo De Acción
The mechanism of action of Fmoc-Phe-Ser(Psi(Me,Me)pro)-OH involves its interaction with specific molecular targets, such as enzymes and receptors. The pseudo-proline moiety plays a crucial role in modulating the peptide’s conformation, thereby influencing its binding affinity and specificity. The compound can inhibit or activate target proteins by mimicking natural substrates or ligands, leading to various biological effects.
Comparación Con Compuestos Similares
Structural and Physicochemical Properties
The pseudoproline moiety [Psi(Me,Me)pro] distinguishes Fmoc-Phe-Ser(Psi(Me,Me)pro)-OH from conventional dipeptides. Key structural comparisons with analogs include:
Key Observations :
- Amino Acid Substitution: Replacing Phe with smaller residues (Ala, Val) reduces molecular weight and alters solubility. For example, Fmoc-Ala-Ser(Psi(Me,Me)pro)-OH is water-soluble with NH₄OH assistance, whereas Phe-containing analogs require organic solvents .
- Pseudoproline Impact: The [Psi(Me,Me)pro] group prevents β-sheet formation, improving synthetic yields in cyclization reactions compared to non-pseudoproline analogs .
Research Findings and Case Studies
Peptide Macrocyclization
In a study by Schmiedeberg and Kessler, this compound enabled >80% cyclization efficiency in uPA-derived peptides, whereas non-pseudoproline analogs failed due to β-sheet aggregation. This highlights its critical role in stabilizing transition states during RCM .
Nanomaterial Templating
This compound templated spherical gold nanoparticles (5–20 nm) under mild conditions, contrasting with Fmoc-Phe-Tyr, which formed rectangular particles (50–100 nm) via stronger reduction .
Actividad Biológica
Fmoc-Phe-Ser(Psi(Me,Me)pro)-OH is a synthetic peptide derivative utilized in various biochemical and pharmaceutical research applications. This compound features a fluorenylmethyloxycarbonyl (Fmoc) protecting group, phenylalanine (Phe), and serine (Ser) residues, along with a unique Psi(Me,MePro) modification that enhances its biological activity. This article explores the biological activity of this compound, focusing on its mechanisms of action, synthesis methods, and relevant case studies.
Chemical Structure and Properties
This compound is characterized by the following properties:
Property | Value |
---|---|
Molecular Formula | C₁₉H₁₉N₃O₄ |
Molecular Weight | 365.44 g/mol |
CAS Number | 878797-01-4 |
Density | 1.3 g/cm³ |
Boiling Point | 683.6 °C at 760 mmHg |
The Psi(Me,MePro) modification introduces steric hindrance that can significantly influence the peptide's conformation and stability, potentially enhancing its biological interactions.
The biological activity of this compound is primarily attributed to its role as a building block in peptide synthesis. The presence of the Psi(Me,MePro) modification disrupts β-sheet structures that are often associated with intermolecular aggregation during peptide chain elongation. This disruption leads to improved solvation and kinetics in peptide assembly, making it easier to synthesize larger peptides through convergent strategies and chemoselective ligation techniques .
The mechanism of action involves interaction with specific molecular targets such as enzymes or receptors. The modification can enhance binding affinity and resistance to enzymatic degradation, prolonging the activity of the synthesized peptides .
Case Studies
-
Synthesis of Human Growth Hormone (hGH) Derivatives :
A study demonstrated the successful incorporation of this compound into hGH-derived peptides. The use of this compound facilitated the synthesis of difficult peptide sequences by improving coupling efficiency and reducing aggregation during synthesis. This was evidenced by quantitative incorporation rates when using various coupling reagents like DIC-OxymaPure . -
Peptide Therapeutics Development :
Research has shown that peptides synthesized using this compound exhibit enhanced stability and bioactivity. For instance, the incorporation of this compound into therapeutic peptides has been linked to improved pharmacokinetic properties and increased efficacy in targeting specific biological pathways . -
Protein-Protein Interaction Studies :
This compound has been employed in studies investigating protein-protein interactions. Its structural properties allow for better modeling of interactions within biological systems, providing insights into mechanisms underlying various diseases .
Synthesis Methods
The synthesis of this compound typically follows solid-phase peptide synthesis (SPPS). The process involves:
- Attachment to Resin : The first amino acid is attached to a resin.
- Sequential Addition : Protected amino acids are sequentially added using the Fmoc protection strategy.
- Deprotection : Removal of the Fmoc group using piperidine.
- Coupling Reactions : Formation of peptide bonds with other amino acids or peptides using reagents like DIC and HOBt.
This method allows for high yield and purity while enabling customization of peptide sequences .
Q & A
Basic Research Questions
Q. What is the role of the pseudoproline moiety [Ser(Psi(Me,Me)pro)] in Fmoc-Phe-Ser(Psi(Me,Me)pro)-OH during peptide synthesis?
The pseudoproline moiety disrupts secondary structures (e.g., β-sheets or β-hairpins) during solid-phase peptide synthesis (SPPS), preventing aggregation and improving solubility. This is achieved by introducing a reversible cis-amide bond, which reduces steric hindrance and facilitates chain elongation . After synthesis, the pseudoproline is cleaved under acidic conditions (e.g., trifluoroacetic acid) to regenerate the native serine residue.
Q. What are the recommended storage and handling protocols for this compound?
- Storage: Store the compound in powder form at -20°C for up to 3 years or at 4°C for 2 years. Solutions in organic solvents (e.g., DMF or DMSO) should be kept at -80°C for 6 months or -20°C for 1 month .
- Handling: Use protective gloves, eye protection, and avoid inhalation of dust. Ensure proper ventilation in the workspace .
Q. How does the presence of pseudoproline derivatives impact peptide solubility during synthesis?
Pseudoprolines like Ser(Psi(Me,Me)pro) enhance solubility by disrupting hydrophobic interactions and hydrogen bonding that lead to aggregation. This is critical for synthesizing long or structurally complex peptides. Solubility can be further optimized by adjusting solvent systems (e.g., using DMF with 0.1% HOBt/DIPEA) .
Q. What analytical methods are used to confirm the structural integrity of this compound?
- HPLC: Assess purity (>98% recommended for SPPS) .
- NMR (1H and 13C): Verify backbone conformation and pseudoproline ring structure .
- Mass spectrometry (ESI-MS or MALDI-TOF): Confirm molecular weight and absence of truncation products .
Advanced Research Questions
Q. How can this compound be applied to improve cyclization efficiency in macrocyclic peptides?
Pseudoprolines facilitate ring-closing metathesis (RCM) or lactamization by reducing steric clashes and stabilizing transition states. For example, in cyclizing uPA-derived peptides, substituting Ser6 with Ser(Psi(Me,Me)pro) increased RCM yields from <20% to >60% by preventing β-sheet formation . Key steps:
Design: Replace aggregation-prone residues (e.g., Ser/Thr) with pseudoproline derivatives.
Optimization: Screen ring sizes (8–12 atoms) and reaction conditions (Grubbs catalyst, 25–40°C).
Validation: Use circular dichroism (CD) to confirm secondary structure disruption .
Q. What experimental strategies resolve contradictions in pseudoproline-mediated peptide cyclization data?
Discrepancies in cyclization efficiency may arise from:
- Sequence context: Adjacent residues (e.g., bulky aromatic groups) may sterically hinder cyclization. Replace with flexible linkers (e.g., Gly) .
- Protecting group incompatibility: Use orthogonal protecting groups (e.g., 2,4-dimethoxybenzyl) to prevent side reactions .
- Incomplete pseudoproline cleavage: Verify cleavage efficiency via LC-MS and adjust TFA exposure time .
Q. How do pseudoprolines influence the conformational dynamics of peptide backbones in solution?
Pseudoprolines introduce a kink in the peptide backbone, stabilizing cis-amide bonds and altering conformational equilibria. Techniques to study this include:
- Molecular dynamics (MD) simulations: Model backbone flexibility and hydrogen-bonding networks.
- Nuclear Overhauser effect (NOE) NMR: Map interproton distances to validate predicted conformations .
Q. What are the limitations of using this compound in automated SPPS?
- Cleavage variability: Over-acidolysis can degrade sensitive residues (e.g., Trp). Optimize cleavage cocktails (e.g., TFA:H2O:triisopropylsilane = 95:2.5:2.5) .
- Coupling inefficiency: Bulky pseudoprolines may slow acylation. Use double coupling protocols with HATU/DIPEA activation .
Q. Methodological Insights Table
Q. Citations
Propiedades
IUPAC Name |
(4S)-3-[(2S)-2-(9H-fluoren-9-ylmethoxycarbonylamino)-3-phenylpropanoyl]-2,2-dimethyl-1,3-oxazolidine-4-carboxylic acid | |
---|---|---|
Source | PubChem | |
URL | https://pubchem.ncbi.nlm.nih.gov | |
Description | Data deposited in or computed by PubChem | |
InChI |
InChI=1S/C30H30N2O6/c1-30(2)32(26(18-38-30)28(34)35)27(33)25(16-19-10-4-3-5-11-19)31-29(36)37-17-24-22-14-8-6-12-20(22)21-13-7-9-15-23(21)24/h3-15,24-26H,16-18H2,1-2H3,(H,31,36)(H,34,35)/t25-,26-/m0/s1 | |
Source | PubChem | |
URL | https://pubchem.ncbi.nlm.nih.gov | |
Description | Data deposited in or computed by PubChem | |
InChI Key |
PQJUKTSKOYOXMZ-UIOOFZCWSA-N | |
Source | PubChem | |
URL | https://pubchem.ncbi.nlm.nih.gov | |
Description | Data deposited in or computed by PubChem | |
Canonical SMILES |
CC1(N(C(CO1)C(=O)O)C(=O)C(CC2=CC=CC=C2)NC(=O)OCC3C4=CC=CC=C4C5=CC=CC=C35)C | |
Source | PubChem | |
URL | https://pubchem.ncbi.nlm.nih.gov | |
Description | Data deposited in or computed by PubChem | |
Isomeric SMILES |
CC1(N([C@@H](CO1)C(=O)O)C(=O)[C@H](CC2=CC=CC=C2)NC(=O)OCC3C4=CC=CC=C4C5=CC=CC=C35)C | |
Source | PubChem | |
URL | https://pubchem.ncbi.nlm.nih.gov | |
Description | Data deposited in or computed by PubChem | |
Molecular Formula |
C30H30N2O6 | |
Source | PubChem | |
URL | https://pubchem.ncbi.nlm.nih.gov | |
Description | Data deposited in or computed by PubChem | |
Molecular Weight |
514.6 g/mol | |
Source | PubChem | |
URL | https://pubchem.ncbi.nlm.nih.gov | |
Description | Data deposited in or computed by PubChem | |
Descargo de responsabilidad e información sobre productos de investigación in vitro
Tenga en cuenta que todos los artículos e información de productos presentados en BenchChem están destinados únicamente con fines informativos. Los productos disponibles para la compra en BenchChem están diseñados específicamente para estudios in vitro, que se realizan fuera de organismos vivos. Los estudios in vitro, derivados del término latino "in vidrio", involucran experimentos realizados en entornos de laboratorio controlados utilizando células o tejidos. Es importante tener en cuenta que estos productos no se clasifican como medicamentos y no han recibido la aprobación de la FDA para la prevención, tratamiento o cura de ninguna condición médica, dolencia o enfermedad. Debemos enfatizar que cualquier forma de introducción corporal de estos productos en humanos o animales está estrictamente prohibida por ley. Es esencial adherirse a estas pautas para garantizar el cumplimiento de los estándares legales y éticos en la investigación y experimentación.