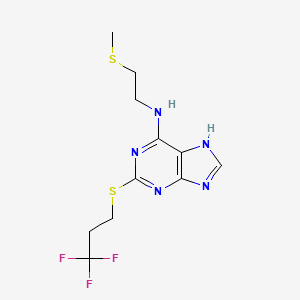
Cangrelor Impurity 8
Descripción general
Descripción
an impurity of Cangrelor
Mecanismo De Acción
Target of Action
Cangrelor Impurity 8, like Cangrelor, primarily targets the P2Y12 platelet receptor . This receptor plays a crucial role in platelet aggregation, a process that contributes to the formation of blood clots. By antagonizing this receptor, this compound can inhibit platelet aggregation .
Mode of Action
This compound acts as a selective, reversible antagonist of the P2Y12 platelet receptor . It inhibits ADP-induced platelet aggregation, which is typically triggered by damaged blood vessels, red blood cells, and/or platelets . This interaction with its target leads to changes in platelet activity, reducing their ability to form clots .
Biochemical Pathways
The primary biochemical pathway affected by this compound is the platelet activation pathway . By inhibiting the P2Y12 receptor, it prevents ADP from stimulating platelet activity. This results in a decrease in platelet aggregation, thereby reducing the risk of clot formation .
Pharmacokinetics
This compound, similar to Cangrelor, is characterized by its rapid onset and offset of action . Its short half-life of approximately 3-6 minutes results in a rapid offset of antiplatelet effect .
Result of Action
The molecular and cellular effects of this compound’s action primarily involve the inhibition of platelet aggregation . This results in a reduced risk of periprocedural myocardial infarction (MI), repeat coronary revascularization, and stent thrombosis . In clinical trials, Cangrelor has been shown to significantly reduce these risks compared with clopidogrel .
Análisis Bioquímico
Cellular Effects
Cangrelor, the parent compound, has been shown to reduce myocardial infarction size in animal models
Molecular Mechanism
Its parent compound, Cangrelor, is a selective, reversible, P2Y12 platelet receptor antagonist which inhibits ADP platelet aggregation
Dosage Effects in Animal Models
Studies on Cangrelor have shown that it can prevent thrombosis in animal models of extracorporeal life support
Metabolic Pathways
Cangrelor, the parent compound, is deactivated rapidly in the circulation by dephosphorylation to its primary metabolite
Propiedades
IUPAC Name |
N-(2-methylsulfanylethyl)-2-(3,3,3-trifluoropropylsulfanyl)-7H-purin-6-amine | |
---|---|---|
Source | PubChem | |
URL | https://pubchem.ncbi.nlm.nih.gov | |
Description | Data deposited in or computed by PubChem | |
InChI |
InChI=1S/C11H14F3N5S2/c1-20-5-3-15-8-7-9(17-6-16-7)19-10(18-8)21-4-2-11(12,13)14/h6H,2-5H2,1H3,(H2,15,16,17,18,19) | |
Source | PubChem | |
URL | https://pubchem.ncbi.nlm.nih.gov | |
Description | Data deposited in or computed by PubChem | |
InChI Key |
JNDPACLVBHNENT-UHFFFAOYSA-N | |
Source | PubChem | |
URL | https://pubchem.ncbi.nlm.nih.gov | |
Description | Data deposited in or computed by PubChem | |
Canonical SMILES |
CSCCNC1=NC(=NC2=C1NC=N2)SCCC(F)(F)F | |
Source | PubChem | |
URL | https://pubchem.ncbi.nlm.nih.gov | |
Description | Data deposited in or computed by PubChem | |
Molecular Formula |
C11H14F3N5S2 | |
Source | PubChem | |
URL | https://pubchem.ncbi.nlm.nih.gov | |
Description | Data deposited in or computed by PubChem | |
Molecular Weight |
337.4 g/mol | |
Source | PubChem | |
URL | https://pubchem.ncbi.nlm.nih.gov | |
Description | Data deposited in or computed by PubChem | |
CAS No. |
1830294-25-1 | |
Record name | 9H-Purin-6-amine, N-(2-(methylthio)ethyl)-2-((3,3,3-trifluoropropyl)thio)- | |
Source | ChemIDplus | |
URL | https://pubchem.ncbi.nlm.nih.gov/substance/?source=chemidplus&sourceid=1830294251 | |
Description | ChemIDplus is a free, web search system that provides access to the structure and nomenclature authority files used for the identification of chemical substances cited in National Library of Medicine (NLM) databases, including the TOXNET system. | |
Record name | 9H-PURIN-6-AMINE, N-(2-(METHYLTHIO)ETHYL)-2-((3,3,3-TRIFLUOROPROPYL)THIO)- | |
Source | FDA Global Substance Registration System (GSRS) | |
URL | https://gsrs.ncats.nih.gov/ginas/app/beta/substances/4N6RMF8XHB | |
Description | The FDA Global Substance Registration System (GSRS) enables the efficient and accurate exchange of information on what substances are in regulated products. Instead of relying on names, which vary across regulatory domains, countries, and regions, the GSRS knowledge base makes it possible for substances to be defined by standardized, scientific descriptions. | |
Explanation | Unless otherwise noted, the contents of the FDA website (www.fda.gov), both text and graphics, are not copyrighted. They are in the public domain and may be republished, reprinted and otherwise used freely by anyone without the need to obtain permission from FDA. Credit to the U.S. Food and Drug Administration as the source is appreciated but not required. | |
Retrosynthesis Analysis
AI-Powered Synthesis Planning: Our tool employs the Template_relevance Pistachio, Template_relevance Bkms_metabolic, Template_relevance Pistachio_ringbreaker, Template_relevance Reaxys, Template_relevance Reaxys_biocatalysis model, leveraging a vast database of chemical reactions to predict feasible synthetic routes.
One-Step Synthesis Focus: Specifically designed for one-step synthesis, it provides concise and direct routes for your target compounds, streamlining the synthesis process.
Accurate Predictions: Utilizing the extensive PISTACHIO, BKMS_METABOLIC, PISTACHIO_RINGBREAKER, REAXYS, REAXYS_BIOCATALYSIS database, our tool offers high-accuracy predictions, reflecting the latest in chemical research and data.
Strategy Settings
Precursor scoring | Relevance Heuristic |
---|---|
Min. plausibility | 0.01 |
Model | Template_relevance |
Template Set | Pistachio/Bkms_metabolic/Pistachio_ringbreaker/Reaxys/Reaxys_biocatalysis |
Top-N result to add to graph | 6 |
Feasible Synthetic Routes
Descargo de responsabilidad e información sobre productos de investigación in vitro
Tenga en cuenta que todos los artículos e información de productos presentados en BenchChem están destinados únicamente con fines informativos. Los productos disponibles para la compra en BenchChem están diseñados específicamente para estudios in vitro, que se realizan fuera de organismos vivos. Los estudios in vitro, derivados del término latino "in vidrio", involucran experimentos realizados en entornos de laboratorio controlados utilizando células o tejidos. Es importante tener en cuenta que estos productos no se clasifican como medicamentos y no han recibido la aprobación de la FDA para la prevención, tratamiento o cura de ninguna condición médica, dolencia o enfermedad. Debemos enfatizar que cualquier forma de introducción corporal de estos productos en humanos o animales está estrictamente prohibida por ley. Es esencial adherirse a estas pautas para garantizar el cumplimiento de los estándares legales y éticos en la investigación y experimentación.