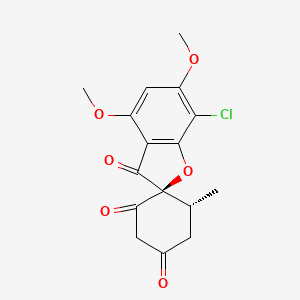
Ácido griseofulvico
Descripción general
Descripción
El ácido griseofúlvico es un metabolito del agente antifúngico griseofulvina. Es conocido por su papel en la inducción de la agregación de proteínas y la polimerización de tubulina en ensayos libres de células . El compuesto tiene una fórmula molecular de C₁₆H₁₅ClO₆ y un peso molecular de 338,74 g/mol .
Aplicaciones Científicas De Investigación
El ácido griseofúlvico tiene una amplia gama de aplicaciones en la investigación científica:
Química: Utilizado como compuesto modelo para estudiar reacciones de oxidación y reducción.
Biología: Investigado por su papel en la agregación de proteínas y la polimerización de tubulina.
Medicina: Explorado por sus posibles propiedades antifúngicas y como metabolito de griseofulvina.
Industria: Utilizado en el desarrollo de nuevos agentes antifúngicos y otros fármacos
Análisis Bioquímico
Biochemical Properties
Griseofulvic Acid plays a significant role in biochemical reactions, particularly in the process of protein aggregation and tubulin polymerization
Molecular Mechanism
Its ability to induce protein aggregation and tubulin polymerization suggests that it may exert its effects at the molecular level through binding interactions with these biomolecules .
Métodos De Preparación
Rutas Sintéticas y Condiciones de Reacción
El ácido griseofúlvico se puede sintetizar a través de diversas reacciones químicas que involucran griseofulvina. Un método común implica la oxidación de griseofulvina utilizando agentes oxidantes específicos en condiciones controladas. La reacción generalmente requiere un solvente como metanol o etanol y se lleva a cabo a temperatura ambiente .
Métodos de Producción Industrial
La producción industrial de ácido griseofúlvico a menudo implica procesos de oxidación a gran escala. Estos procesos se optimizan para el rendimiento y la pureza, utilizando técnicas avanzadas como reactores de flujo continuo y sistemas de control automatizados para mantener condiciones de reacción consistentes .
Análisis De Reacciones Químicas
Tipos de Reacciones
El ácido griseofúlvico experimenta varios tipos de reacciones químicas, que incluyen:
Oxidación: Conversión de griseofulvina a ácido griseofúlvico.
Reducción: Posible reducción del ácido griseofúlvico a otros metabolitos.
Sustitución: Posibles reacciones de sustitución que involucran el átomo de cloro en su estructura.
Reactivos y Condiciones Comunes
Agentes Oxidantes: Peróxido de hidrógeno, permanganato de potasio.
Agentes Reductores: Borohidruro de sodio, hidruro de aluminio y litio.
Solventes: Metanol, etanol, diclorometano.
Principales Productos
El producto principal de la oxidación de griseofulvina es el ácido griseofúlvico. Otros productos potenciales incluyen varios derivados reducidos o sustituidos, dependiendo de los reactivos y condiciones específicos utilizados .
Mecanismo De Acción
El ácido griseofúlvico ejerce sus efectos principalmente a través de la inducción de la agregación de proteínas y la polimerización de tubulina. Interactúa con los microtúbulos, promoviendo su ensamblaje y estabilización. Esta acción interrumpe las funciones celulares normales, lo que lleva a efectos antifúngicos .
Comparación Con Compuestos Similares
Compuestos Similares
Griseofulvina: El compuesto principal del que se deriva el ácido griseofúlvico.
Ácido Fúlvico: Otro compuesto con características estructurales similares pero diferentes actividades biológicas.
Ácido Clorogénico: Comparte algunas propiedades químicas pero tiene efectos biológicos distintos
Unicidad
El ácido griseofúlvico es único debido a su papel específico como metabolito de la griseofulvina y su capacidad para inducir la agregación de proteínas y la polimerización de tubulina. Esto lo convierte en un compuesto valioso para estudiar estos procesos y desarrollar nuevos agentes antifúngicos .
Actividad Biológica
Griseofulvic acid, a derivative of griseofulvin, has garnered attention for its diverse biological activities, particularly in the fields of antifungal, anticancer, and antiviral research. This article synthesizes current knowledge regarding the biological activity of griseofulvic acid, supported by data tables and case studies.
Overview of Griseofulvic Acid
Griseofulvin is an antifungal agent derived from the mold Penicillium griseofulvum, primarily used to treat dermatophyte infections. Griseofulvic acid is synthesized from griseofulvin through various chemical processes and has shown potential in multiple therapeutic areas beyond its antifungal properties.
Antifungal Activity
Griseofulvic acid exhibits significant antifungal activity against a variety of fungal pathogens. Its mechanism involves disruption of fungal cell division and interference with mitotic spindle formation, similar to its parent compound, griseofulvin.
Table 1: Antifungal Activity of Griseofulvic Acid
Fungal Strain | Minimum Inhibitory Concentration (MIC) | Reference |
---|---|---|
Candida albicans | 8 µg/mL | |
Aspergillus niger | 16 µg/mL | |
Trichophyton rubrum | 4 µg/mL |
Anticancer Properties
Recent studies have highlighted the anticancer potential of griseofulvic acid. It has been shown to inhibit the proliferation of various cancer cell lines by affecting microtubule dynamics during mitosis.
Case Studies:
- Colorectal Cancer : Griseofulvic acid demonstrated significant inhibition of HT-29 and COLO-205 cell lines with an IC50 value of 20 µM after 24 hours of treatment, indicating its potential as a therapeutic agent in colorectal cancer management .
- Liver Cancer : In Hep G2 cells, treatment with griseofulvic acid resulted in reduced cell viability and induced apoptosis through upregulation of pro-apoptotic factors such as Bax and p53 while downregulating anti-apoptotic factors like Bcl-2 .
Table 2: Anticancer Effects of Griseofulvic Acid
Cancer Type | Cell Line | IC50 (µM) | Mechanism of Action |
---|---|---|---|
Colorectal Cancer | HT-29 | 20 | Microtubule disruption |
Liver Cancer | Hep G2 | 25 | Apoptosis induction |
Cervical Cancer | HeLa | 35 | Cell cycle arrest |
Antiviral Activity
Griseofulvic acid has also been investigated for its antiviral properties, particularly against SARS-CoV-2. Molecular docking studies revealed that it binds effectively to the main protease and RNA-dependent RNA polymerase (RdRp) of the virus, suggesting potential as a therapeutic agent in COVID-19 treatment .
Table 3: Antiviral Activity Against SARS-CoV-2
Target Protein | Binding Affinity (kcal/mol) | Reference |
---|---|---|
Main Protease | -9.5 | |
RNA-dependent RNA Polymerase (RdRp) | -8.7 |
The biological activity of griseofulvic acid can be attributed to several mechanisms:
- Inhibition of Mitosis : By disrupting microtubule formation, it prevents proper cell division in both fungal and cancer cells.
- Apoptosis Induction : It promotes apoptosis in cancer cells by modulating key apoptotic pathways.
- Antimicrobial Properties : Its ability to inhibit carbonic anhydrases in various microorganisms offers a novel approach to combatting drug-resistant strains .
Propiedades
IUPAC Name |
(2S,5'R)-7-chloro-4,6-dimethoxy-5'-methylspiro[1-benzofuran-2,4'-cyclohexane]-1',3,3'-trione | |
---|---|---|
Source | PubChem | |
URL | https://pubchem.ncbi.nlm.nih.gov | |
Description | Data deposited in or computed by PubChem | |
InChI |
InChI=1S/C16H15ClO6/c1-7-4-8(18)5-11(19)16(7)15(20)12-9(21-2)6-10(22-3)13(17)14(12)23-16/h6-7H,4-5H2,1-3H3/t7-,16+/m1/s1 | |
Source | PubChem | |
URL | https://pubchem.ncbi.nlm.nih.gov | |
Description | Data deposited in or computed by PubChem | |
InChI Key |
YIQRSAKVWZVLDN-QZTNRIJFSA-N | |
Source | PubChem | |
URL | https://pubchem.ncbi.nlm.nih.gov | |
Description | Data deposited in or computed by PubChem | |
Canonical SMILES |
CC1CC(=O)CC(=O)C12C(=O)C3=C(O2)C(=C(C=C3OC)OC)Cl | |
Source | PubChem | |
URL | https://pubchem.ncbi.nlm.nih.gov | |
Description | Data deposited in or computed by PubChem | |
Isomeric SMILES |
C[C@@H]1CC(=O)CC(=O)[C@]12C(=O)C3=C(O2)C(=C(C=C3OC)OC)Cl | |
Source | PubChem | |
URL | https://pubchem.ncbi.nlm.nih.gov | |
Description | Data deposited in or computed by PubChem | |
Molecular Formula |
C16H15ClO6 | |
Source | PubChem | |
URL | https://pubchem.ncbi.nlm.nih.gov | |
Description | Data deposited in or computed by PubChem | |
Molecular Weight |
338.74 g/mol | |
Source | PubChem | |
URL | https://pubchem.ncbi.nlm.nih.gov | |
Description | Data deposited in or computed by PubChem | |
CAS No. |
469-54-5 | |
Record name | Griseofulvic acid | |
Source | ChemIDplus | |
URL | https://pubchem.ncbi.nlm.nih.gov/substance/?source=chemidplus&sourceid=0000469545 | |
Description | ChemIDplus is a free, web search system that provides access to the structure and nomenclature authority files used for the identification of chemical substances cited in National Library of Medicine (NLM) databases, including the TOXNET system. | |
Record name | GRISEOFULVIC ACID | |
Source | FDA Global Substance Registration System (GSRS) | |
URL | https://gsrs.ncats.nih.gov/ginas/app/beta/substances/DMG8UC5TE5 | |
Description | The FDA Global Substance Registration System (GSRS) enables the efficient and accurate exchange of information on what substances are in regulated products. Instead of relying on names, which vary across regulatory domains, countries, and regions, the GSRS knowledge base makes it possible for substances to be defined by standardized, scientific descriptions. | |
Explanation | Unless otherwise noted, the contents of the FDA website (www.fda.gov), both text and graphics, are not copyrighted. They are in the public domain and may be republished, reprinted and otherwise used freely by anyone without the need to obtain permission from FDA. Credit to the U.S. Food and Drug Administration as the source is appreciated but not required. | |
Descargo de responsabilidad e información sobre productos de investigación in vitro
Tenga en cuenta que todos los artículos e información de productos presentados en BenchChem están destinados únicamente con fines informativos. Los productos disponibles para la compra en BenchChem están diseñados específicamente para estudios in vitro, que se realizan fuera de organismos vivos. Los estudios in vitro, derivados del término latino "in vidrio", involucran experimentos realizados en entornos de laboratorio controlados utilizando células o tejidos. Es importante tener en cuenta que estos productos no se clasifican como medicamentos y no han recibido la aprobación de la FDA para la prevención, tratamiento o cura de ninguna condición médica, dolencia o enfermedad. Debemos enfatizar que cualquier forma de introducción corporal de estos productos en humanos o animales está estrictamente prohibida por ley. Es esencial adherirse a estas pautas para garantizar el cumplimiento de los estándares legales y éticos en la investigación y experimentación.