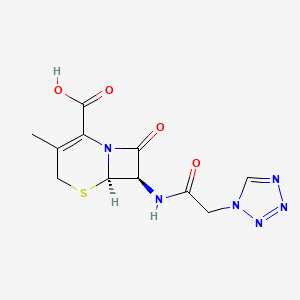
Cefazolin Impurity C
Descripción general
Descripción
Métodos De Preparación
La preparación del Ácido 7-[1-(1H)-Tetrazolylacetamido]desacetoxi-cefalosporánico implica varias rutas sintéticas y condiciones de reacción. Los métodos de producción industrial pueden variar, pero generalmente implican condiciones de reacción controladas para garantizar la pureza y la estabilidad del producto final.
Análisis De Reacciones Químicas
El Ácido 7-[1-(1H)-Tetrazolylacetamido]desacetoxi-cefalosporánico experimenta varios tipos de reacciones químicas, que incluyen:
Oxidación: El compuesto puede oxidarse bajo condiciones específicas para formar diferentes productos de oxidación.
Reducción: Las reacciones de reducción se pueden llevar a cabo utilizando agentes reductores comunes.
Sustitución: El grupo tetrazolylacetamido puede participar en reacciones de sustitución con varios reactivos.
Los reactivos y condiciones comunes utilizados en estas reacciones incluyen agentes oxidantes como el peróxido de hidrógeno, agentes reductores como el borohidruro de sodio y varios nucleófilos para reacciones de sustitución. Los principales productos formados a partir de estas reacciones dependen de las condiciones y los reactivos específicos utilizados .
Aplicaciones Científicas De Investigación
Toxicity Assessments
Recent studies have focused on the toxicological impact of various cefazolin impurities, including Impurity C. Research utilizing zebrafish models has demonstrated that certain structural components within these impurities correlate with specific toxic effects:
- Embryonic Development : Studies indicate that certain impurities can lead to teratogenic effects, including deformities in embryonic development. For instance, the structure-toxicity relationship revealed that specific functional groups within impurities could induce cardiac and neurobehavioral toxicity in zebrafish larvae .
- Cardiotoxicity : The cardiotoxic effects observed with cefazolin impurities are particularly concerning. Impurity G (7-aminocephalosporanic acid) exhibited significant cardiac toxicity, leading to atrioventricular conduction blocks in experimental models .
The analytical techniques employed to identify and quantify cefazolin impurities include:
- High-Performance Liquid Chromatography (HPLC) : This method is essential for separating and characterizing the various degradation products and impurities present in cefazolin formulations. Studies have successfully isolated unknown impurities using gradient reversed-phase HPLC coupled with mass spectrometry (LC-MS/MS) to elucidate their structures .
- Mass Spectrometry : LC-MS/MS provides detailed fragmentation patterns that aid in identifying the molecular structure of impurities. For example, specific mass spectral fragments corresponding to degradation pathways have been documented, enhancing our understanding of how these impurities form during storage or processing .
Table 2: Analytical Techniques for Cefazolin Impurities
Technique | Purpose |
---|---|
HPLC | Separation and quantification |
LC-MS/MS | Structural elucidation |
Nuclear Magnetic Resonance (NMR) | Confirmation of molecular structure |
Case Studies
Several case studies have provided insights into the implications of cefazolin impurities:
- Case Study 1 : A study assessed the embryotoxic effects of cefazolin sodium impurities using zebrafish embryos. It was found that exposure to impurity F resulted in significant developmental abnormalities compared to controls .
- Case Study 2 : Another research effort focused on the degradation pathways leading to impurity formation in cefazolin sodium. The study utilized HPLC and LC-MS/MS to track the stability of cefazolin under various conditions, revealing critical insights into how environmental factors influence impurity levels .
Regulatory Considerations
The presence of impurities like Cefazolin Impurity C raises important regulatory questions regarding drug safety standards. Regulatory agencies require comprehensive impurity profiling as part of quality control measures to ensure patient safety.
Mecanismo De Acción
El mecanismo de acción del Ácido 7-[1-(1H)-Tetrazolylacetamido]desacetoxi-cefalosporánico implica su interacción con objetivos y vías moleculares específicos. El compuesto puede ejercer sus efectos uniéndose a enzimas bacterianas e inhibiendo su actividad, lo que interrumpe la síntesis de la pared celular bacteriana. Este mecanismo es similar al de otros antibióticos cefalosporínicos, que se dirigen a las proteínas de unión a la penicilina e interfieren con la reticulación del peptidoglicano en las paredes celulares bacterianas .
Comparación Con Compuestos Similares
El Ácido 7-[1-(1H)-Tetrazolylacetamido]desacetoxi-cefalosporánico se puede comparar con otros compuestos similares, como:
Cefazolina: Un antibiótico cefalosporínico ampliamente utilizado con una estructura central similar pero diferentes sustituyentes.
Cefuroxima: Otro antibiótico cefalosporínico con un espectro de actividad y una estructura química diferentes.
Ceftriaxona: Conocida por su actividad de amplio espectro y diferentes propiedades farmacocinéticas.
La singularidad del Ácido 7-[1-(1H)-Tetrazolylacetamido]desacetoxi-cefalosporánico radica en su grupo tetrazolylacetamido específico, que puede impartir propiedades químicas y biológicas distintas en comparación con otras cefalosporinas .
Actividad Biológica
Cefazolin, a first-generation cephalosporin antibiotic, is primarily known for its effectiveness against a broad spectrum of gram-positive and some gram-negative bacteria. However, the presence of impurities, particularly Cefazolin Impurity C, raises important questions regarding its biological activity and safety profile. This article delves into the biological activity of this compound, highlighting relevant research findings, case studies, and data tables.
Overview of Cefazolin and Its Impurities
Cefazolin is widely used in clinical settings for treating various bacterial infections. It functions by inhibiting bacterial cell wall synthesis through binding to penicillin-binding proteins (PBPs) within the bacterial cell wall . Despite its efficacy, the presence of degradation products and impurities can influence its pharmacological properties and safety.
Impurity Characterization
This compound is one of several degradation products that can arise from the chemical instability of cefazolin under certain conditions. Research indicates that impurities can impact the drug's safety and efficacy. For example, a study identified several degradation impurities in cefazolin sodium using high-performance liquid chromatography (HPLC), including Impurity C .
Anti-inflammatory Effects
Recent studies have demonstrated that cefazolin exhibits anti-inflammatory properties. In vitro experiments showed that cefazolin could reduce the proliferation of peripheral blood mononuclear cells (PBMCs) when stimulated by various interleukins (IL-2, IL-4, IL-15) . Notably, it inhibited the production of pro-inflammatory cytokines such as IFN-γ, IL-17, and TNF-α. This suggests that cefazolin may modulate immune responses beyond its antibacterial action.
Toxicity Studies
A significant aspect of understanding this compound involves assessing its toxicity. A study conducted on zebrafish embryos evaluated the embryotoxicity and developmental effects of multiple cefazolin impurities, including Impurity C. The results indicated varying degrees of toxicity among different impurities, with some showing significant effects on embryo development and motor function .
Case Studies
Case Study 1: Complicated Urinary Tract Infections (cUTIs)
In a clinical setting, cefazolin was reported as an effective treatment for cUTIs caused by susceptible strains of Escherichia coli. The case highlighted the importance of accurately reporting cefazolin susceptibility to guide treatment decisions effectively .
Case Study 2: Systemic Infections
Another case documented the successful use of cefazolin in treating systemic infections due to Klebsiella pneumoniae. The patient's response to treatment was monitored closely to evaluate the drug's efficacy against this pathogen .
Data Tables
The following table summarizes key findings related to the biological activity and toxicity of this compound:
Parameter | Cefazolin | This compound |
---|---|---|
Mechanism of Action | Inhibition of cell wall synthesis | Potential modulation of immune response |
Toxicity Level | Low | Moderate (based on zebrafish studies) |
Efficacy against Gram-positive | High | Unknown |
Efficacy against Gram-negative | Moderate | Unknown |
Anti-inflammatory Activity | Present | Unknown |
Propiedades
IUPAC Name |
(6R,7R)-3-methyl-8-oxo-7-[[2-(tetrazol-1-yl)acetyl]amino]-5-thia-1-azabicyclo[4.2.0]oct-2-ene-2-carboxylic acid | |
---|---|---|
Source | PubChem | |
URL | https://pubchem.ncbi.nlm.nih.gov | |
Description | Data deposited in or computed by PubChem | |
InChI |
InChI=1S/C11H12N6O4S/c1-5-3-22-10-7(9(19)17(10)8(5)11(20)21)13-6(18)2-16-4-12-14-15-16/h4,7,10H,2-3H2,1H3,(H,13,18)(H,20,21)/t7-,10-/m1/s1 | |
Source | PubChem | |
URL | https://pubchem.ncbi.nlm.nih.gov | |
Description | Data deposited in or computed by PubChem | |
InChI Key |
DGYURGWZOSQCFG-GMSGAONNSA-N | |
Source | PubChem | |
URL | https://pubchem.ncbi.nlm.nih.gov | |
Description | Data deposited in or computed by PubChem | |
Canonical SMILES |
CC1=C(N2C(C(C2=O)NC(=O)CN3C=NN=N3)SC1)C(=O)O | |
Source | PubChem | |
URL | https://pubchem.ncbi.nlm.nih.gov | |
Description | Data deposited in or computed by PubChem | |
Isomeric SMILES |
CC1=C(N2[C@@H]([C@@H](C2=O)NC(=O)CN3C=NN=N3)SC1)C(=O)O | |
Source | PubChem | |
URL | https://pubchem.ncbi.nlm.nih.gov | |
Description | Data deposited in or computed by PubChem | |
Molecular Formula |
C11H12N6O4S | |
Source | PubChem | |
URL | https://pubchem.ncbi.nlm.nih.gov | |
Description | Data deposited in or computed by PubChem | |
Molecular Weight |
324.32 g/mol | |
Source | PubChem | |
URL | https://pubchem.ncbi.nlm.nih.gov | |
Description | Data deposited in or computed by PubChem | |
CAS No. |
56842-77-4 | |
Record name | (6R,7R)-7-(2-(1H-Tetrazol-1-yl)acetamido)-3-methyl-8-oxo-5-thia-1-azabicyclo(4.2.0)oct-2-ene-2-carboxylic acid | |
Source | ChemIDplus | |
URL | https://pubchem.ncbi.nlm.nih.gov/substance/?source=chemidplus&sourceid=0056842774 | |
Description | ChemIDplus is a free, web search system that provides access to the structure and nomenclature authority files used for the identification of chemical substances cited in National Library of Medicine (NLM) databases, including the TOXNET system. | |
Record name | CEFAZOLIN 3-METHYL ANALOG | |
Source | FDA Global Substance Registration System (GSRS) | |
URL | https://gsrs.ncats.nih.gov/ginas/app/beta/substances/PGB16683CG | |
Description | The FDA Global Substance Registration System (GSRS) enables the efficient and accurate exchange of information on what substances are in regulated products. Instead of relying on names, which vary across regulatory domains, countries, and regions, the GSRS knowledge base makes it possible for substances to be defined by standardized, scientific descriptions. | |
Explanation | Unless otherwise noted, the contents of the FDA website (www.fda.gov), both text and graphics, are not copyrighted. They are in the public domain and may be republished, reprinted and otherwise used freely by anyone without the need to obtain permission from FDA. Credit to the U.S. Food and Drug Administration as the source is appreciated but not required. | |
Retrosynthesis Analysis
AI-Powered Synthesis Planning: Our tool employs the Template_relevance Pistachio, Template_relevance Bkms_metabolic, Template_relevance Pistachio_ringbreaker, Template_relevance Reaxys, Template_relevance Reaxys_biocatalysis model, leveraging a vast database of chemical reactions to predict feasible synthetic routes.
One-Step Synthesis Focus: Specifically designed for one-step synthesis, it provides concise and direct routes for your target compounds, streamlining the synthesis process.
Accurate Predictions: Utilizing the extensive PISTACHIO, BKMS_METABOLIC, PISTACHIO_RINGBREAKER, REAXYS, REAXYS_BIOCATALYSIS database, our tool offers high-accuracy predictions, reflecting the latest in chemical research and data.
Strategy Settings
Precursor scoring | Relevance Heuristic |
---|---|
Min. plausibility | 0.01 |
Model | Template_relevance |
Template Set | Pistachio/Bkms_metabolic/Pistachio_ringbreaker/Reaxys/Reaxys_biocatalysis |
Top-N result to add to graph | 6 |
Feasible Synthetic Routes
Descargo de responsabilidad e información sobre productos de investigación in vitro
Tenga en cuenta que todos los artículos e información de productos presentados en BenchChem están destinados únicamente con fines informativos. Los productos disponibles para la compra en BenchChem están diseñados específicamente para estudios in vitro, que se realizan fuera de organismos vivos. Los estudios in vitro, derivados del término latino "in vidrio", involucran experimentos realizados en entornos de laboratorio controlados utilizando células o tejidos. Es importante tener en cuenta que estos productos no se clasifican como medicamentos y no han recibido la aprobación de la FDA para la prevención, tratamiento o cura de ninguna condición médica, dolencia o enfermedad. Debemos enfatizar que cualquier forma de introducción corporal de estos productos en humanos o animales está estrictamente prohibida por ley. Es esencial adherirse a estas pautas para garantizar el cumplimiento de los estándares legales y éticos en la investigación y experimentación.