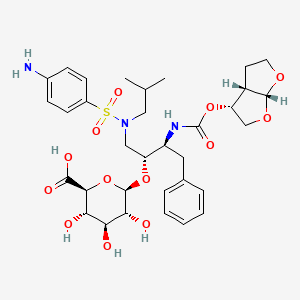
Darunavir O-|A-D-Glucurónido
Descripción general
Descripción
DARUNAVIR O-BETA-D-GLUCURONIDE is a metabolite of darunavir, a protease inhibitor used in the treatment of human immunodeficiency virus (HIV) infection. Darunavir is known for its effectiveness in managing HIV-1 infection, especially in patients with a history of prior antiretroviral therapies . The glucuronide conjugate, DARUNAVIR O-BETA-D-GLUCURONIDE, is formed through the process of glucuronidation, a common phase II metabolic pathway that aids in the elimination of small molecule drugs from the body .
Aplicaciones Científicas De Investigación
Pharmacokinetic Studies
Pharmacokinetic studies are essential for understanding how drugs are absorbed, distributed, metabolized, and excreted in the body. Darunavir O-Beta-D-Glucuronide is formed primarily in the liver through glucuronidation, a process that enhances the solubility and excretion of darunavir.
Key Findings:
- Bioavailability: Darunavir exhibits a bioavailability of 37% to 58% in rats, with higher values observed in dogs (60% to 122%) due to differences in first-pass metabolism .
- Metabolism: The compound undergoes extensive hepatic metabolism via cytochrome P450 enzymes, primarily CYP3A4. The glucuronide metabolite is less pharmacologically active than darunavir itself .
- Elimination: Darunavir O-Beta-D-Glucuronide contributes to the elimination pathway of darunavir, with studies indicating rapid clearance across species .
Drug Interactions
Understanding drug interactions is vital for optimizing therapeutic regimens and minimizing adverse effects. Darunavir O-Beta-D-Glucuronide's role as a metabolite can influence interactions with other medications.
Mechanisms:
- CYP Enzyme Induction: The presence of darunavir can induce CYP enzymes (e.g., CYP2C9, CYP2C19), which may alter the metabolism of co-administered drugs .
- Impact on Etravirine: Studies have shown that co-administration of darunavir with etravirine affects their pharmacokinetic profiles significantly, indicating potential clinical implications for combination therapies .
Therapeutic Applications Beyond HIV
Recent research has begun to explore the potential repurposing of darunavir and its metabolites for other therapeutic applications.
Case Studies:
- Cancer Treatment: Preliminary studies suggest that darunavir may exhibit anti-tumor effects when used in combination with other agents like paclitaxel. This synergy appears to inhibit tumor growth and metastasis in preclinical models .
- Inflammatory Conditions: The modulation of immune responses through glucuronidation pathways may offer insights into treating inflammatory diseases, although more research is needed to substantiate these claims.
Summary Table of Key Pharmacokinetic Parameters
Parameter | Value (Rats) | Value (Dogs) | Value (Humans) |
---|---|---|---|
Bioavailability | 37% - 58% | 60% - 122% | ~95% protein bound |
Metabolic Pathway | CYP3A4 | CYP3A4 | CYP3A4 |
Half-life | Rapid clearance | Moderate | Variable |
Major Metabolite | O-Beta-D-Glucuronide | O-Beta-D-Glucuronide | O-Beta-D-Glucuronide |
Mecanismo De Acción
Target of Action
Darunavir O-|A-D-Glucuronide, also known as Darunavir metabolite M20, primarily targets the HIV-1 protease enzyme . This enzyme plays a crucial role in the life cycle of the HIV virus, as it is necessary for the processing of viral precursor proteins and the maturation of the virus in preparation for infection .
Mode of Action
Darunavir O-|A-D-Glucuronide acts as a nonpeptidic inhibitor of the HIV-1 protease . It lodges itself in the active site of the protease through a number of hydrogen bonds . This binding inhibits the cleavage of viral Gag-Pol polyprotein precursors into individual functional proteins required for infectious HIV . As a result, the formation of mature, infectious viral particles is prevented .
Biochemical Pathways
The inhibition of the HIV-1 protease enzyme by Darunavir O-|A-D-Glucuronide disrupts the normal life cycle of the HIV virus. This disruption prevents the virus from maturing and becoming infectious . The compound is designed to increase interactions with the HIV-1 protease and to be more resistant against HIV-1 protease mutations .
Pharmacokinetics
Darunavir O-|A-D-Glucuronide is heavily oxidized and metabolized by hepatic cytochrome enzymes, mainly CYP3A . In boosted darunavir administration, unchanged darunavir made up 48.8% of the excreted dose in boosted subjects due to inhibition of darunavir metabolism by ritonavir . Unchanged drug in the urine made up 1.2% of the administered dose in volunteers who were unboosted, and 7.7% in boosted volunteers .
Result of Action
The primary result of the action of Darunavir O-|A-D-Glucuronide is the prevention of the maturation of the HIV virus, thereby reducing its infectivity . By inhibiting the HIV-1 protease enzyme, the compound prevents the cleavage of viral Gag-Pol polyprotein precursors into individual functional proteins required for infectious HIV . This leads to the formation of immature, noninfectious viral particles .
Action Environment
The action of Darunavir O-|A-D-Glucuronide can be influenced by various environmental factors. For instance, the compound’s metabolism can be affected by the presence of other drugs that inhibit or induce the CYP3A enzyme . Furthermore, the compound’s action can be enhanced by co-administration with ritonavir, a potent inhibitor of CYP3A, which increases the plasma concentrations of darunavir .
Métodos De Preparación
Synthetic Routes and Reaction Conditions
The synthesis of DARUNAVIR O-BETA-D-GLUCURONIDE involves the glucuronidation of darunavir. This process typically requires the presence of uridine 5′-diphosphoglucuronic acid (UDPGA) as a glucuronic acid donor and is catalyzed by the enzyme UDP-glucuronosyltransferase (UGT). The reaction conditions often include an aqueous buffer system at a physiological pH, and the reaction is carried out at body temperature (37°C) to mimic in vivo conditions .
Industrial Production Methods
Industrial production of DARUNAVIR O-BETA-D-GLUCURONIDE follows similar principles but on a larger scale. The process involves the use of bioreactors where darunavir is incubated with liver microsomes or recombinant UGT enzymes in the presence of UDPGA. The reaction mixture is then purified using chromatographic techniques to isolate the glucuronide conjugate .
Análisis De Reacciones Químicas
Types of Reactions
DARUNAVIR O-BETA-D-GLUCURONIDE primarily undergoes hydrolysis and conjugation reactions. The glucuronide conjugate can be hydrolyzed back to darunavir and glucuronic acid under acidic or enzymatic conditions .
Common Reagents and Conditions
Hydrolysis: Acidic conditions (e.g., hydrochloric acid) or enzymatic hydrolysis using β-glucuronidase.
Conjugation: UDPGA and UGT enzymes in an aqueous buffer system at physiological pH.
Major Products Formed
The major products formed from the hydrolysis of DARUNAVIR O-BETA-D-GLUCURONIDE are darunavir and glucuronic acid .
Comparación Con Compuestos Similares
Similar Compounds
Dolutegravir: Another antiretroviral drug that undergoes glucuronidation to form dolutegravir-glucuronide.
Elvitegravir: An integrase inhibitor that is also metabolized to its glucuronide conjugate.
Rilpivirine: A non-nucleoside reverse transcriptase inhibitor that forms rilpivirine-glucuronide.
Uniqueness
DARUNAVIR O-BETA-D-GLUCURONIDE is unique due to its specific formation from darunavir, a second-generation protease inhibitor designed to combat resistance to standard HIV therapy . Its formation and elimination are crucial for the pharmacokinetics and therapeutic management of darunavir, distinguishing it from other glucuronide conjugates of antiretroviral drugs .
Actividad Biológica
Darunavir O-beta-D-glucuronide is a metabolite of darunavir, an HIV protease inhibitor. Understanding the biological activity of this compound is essential for evaluating its therapeutic efficacy and safety profile. This article reviews the pharmacological properties, metabolic pathways, and biological activities of darunavir O-beta-D-glucuronide, supported by relevant research findings and data tables.
Overview of Darunavir
Darunavir (DRV) is primarily used in the treatment of HIV infections. It works by inhibiting the HIV-1 protease enzyme, which is crucial for viral replication. The compound exhibits high potency against various strains of HIV, including those resistant to other protease inhibitors. Its chemical structure allows it to form strong hydrogen bonds with the active site of the protease, facilitating effective inhibition even in resistant strains .
Metabolism and Formation of Darunavir O-Beta-D-Glucuronide
Darunavir is extensively metabolized in the liver, primarily by cytochrome P450 enzymes (CYP3A4 and CYP2D6). The metabolic process includes glucuronidation, where darunavir is conjugated with glucuronic acid to form darunavir O-beta-D-glucuronide. This metabolite is significant as it may influence the pharmacokinetics and pharmacodynamics of darunavir .
Table 1: Metabolic Pathways of Darunavir
Metabolite | Enzyme Involved | Formation Process |
---|---|---|
Darunavir | CYP3A4 | Hydroxylation |
Darunavir O-beta-D-glucuronide | UGT1A3, UGT1A8 | Glucuronidation |
Biological Activity
Research indicates that darunavir O-beta-D-glucuronide retains some biological activity, although significantly less than its parent compound. The glucuronidation process generally leads to a reduction in the pharmacological activity of drugs. For instance, studies show that while darunavir exhibits potent inhibition against HIV-1 protease with an IC50 value in the low nanomolar range, its glucuronide metabolite displays reduced efficacy .
Case Study: Efficacy Against HIV-1
In a comparative study examining the inhibitory effects of darunavir and its glucuronide metabolite on HIV-1 protease, results demonstrated that darunavir had an IC50 value of approximately 1.87 nM, while darunavir O-beta-D-glucuronide showed a markedly higher IC50 value, indicating reduced potency .
Pharmacokinetics
The pharmacokinetic profile of darunavir O-beta-D-glucuronide suggests that it has a longer half-life compared to unmodified darunavir due to its increased solubility and stability in biological fluids. This could potentially lead to prolonged exposure in systemic circulation; however, its reduced activity necessitates careful consideration when evaluating therapeutic regimens involving darunavir .
Table 2: Pharmacokinetic Properties
Parameter | Darunavir | Darunavir O-Beta-D-Glucuronide |
---|---|---|
Half-Life | ~15 hours | Longer due to glucuronidation |
Bioavailability | ~82% | Unknown |
Clearance | 9.7 L/h | Reduced clearance |
Propiedades
IUPAC Name |
(2S,3S,4S,5R,6R)-6-[(2R,3S)-3-[[(3aS,4R,6aR)-2,3,3a,4,5,6a-hexahydrofuro[2,3-b]furan-4-yl]oxycarbonylamino]-1-[(4-aminophenyl)sulfonyl-(2-methylpropyl)amino]-4-phenylbutan-2-yl]oxy-3,4,5-trihydroxyoxane-2-carboxylic acid | |
---|---|---|
Source | PubChem | |
URL | https://pubchem.ncbi.nlm.nih.gov | |
Description | Data deposited in or computed by PubChem | |
InChI |
InChI=1S/C33H45N3O13S/c1-18(2)15-36(50(43,44)21-10-8-20(34)9-11-21)16-24(47-32-28(39)26(37)27(38)29(49-32)30(40)41)23(14-19-6-4-3-5-7-19)35-33(42)48-25-17-46-31-22(25)12-13-45-31/h3-11,18,22-29,31-32,37-39H,12-17,34H2,1-2H3,(H,35,42)(H,40,41)/t22-,23-,24+,25-,26-,27-,28+,29-,31+,32+/m0/s1 | |
Source | PubChem | |
URL | https://pubchem.ncbi.nlm.nih.gov | |
Description | Data deposited in or computed by PubChem | |
InChI Key |
QZOVHLAWBUMQFG-XXNFXRIYSA-N | |
Source | PubChem | |
URL | https://pubchem.ncbi.nlm.nih.gov | |
Description | Data deposited in or computed by PubChem | |
Canonical SMILES |
CC(C)CN(CC(C(CC1=CC=CC=C1)NC(=O)OC2COC3C2CCO3)OC4C(C(C(C(O4)C(=O)O)O)O)O)S(=O)(=O)C5=CC=C(C=C5)N | |
Source | PubChem | |
URL | https://pubchem.ncbi.nlm.nih.gov | |
Description | Data deposited in or computed by PubChem | |
Isomeric SMILES |
CC(C)CN(C[C@H]([C@H](CC1=CC=CC=C1)NC(=O)O[C@H]2CO[C@@H]3[C@H]2CCO3)O[C@H]4[C@@H]([C@H]([C@@H]([C@H](O4)C(=O)O)O)O)O)S(=O)(=O)C5=CC=C(C=C5)N | |
Source | PubChem | |
URL | https://pubchem.ncbi.nlm.nih.gov | |
Description | Data deposited in or computed by PubChem | |
Molecular Formula |
C33H45N3O13S | |
Source | PubChem | |
URL | https://pubchem.ncbi.nlm.nih.gov | |
Description | Data deposited in or computed by PubChem | |
DSSTOX Substance ID |
DTXSID50747418 | |
Record name | (2R,3S)-1-[(4-Aminobenzene-1-sulfonyl)(2-methylpropyl)amino]-3-[({[(3R,3aS,6aR)-hexahydrofuro[2,3-b]furan-3-yl]oxy}carbonyl)amino]-4-phenylbutan-2-yl beta-D-glucopyranosiduronic acid | |
Source | EPA DSSTox | |
URL | https://comptox.epa.gov/dashboard/DTXSID50747418 | |
Description | DSSTox provides a high quality public chemistry resource for supporting improved predictive toxicology. | |
Molecular Weight |
723.8 g/mol | |
Source | PubChem | |
URL | https://pubchem.ncbi.nlm.nih.gov | |
Description | Data deposited in or computed by PubChem | |
CAS No. |
1159613-27-0 | |
Record name | Darunavir metabolite M20 | |
Source | ChemIDplus | |
URL | https://pubchem.ncbi.nlm.nih.gov/substance/?source=chemidplus&sourceid=1159613270 | |
Description | ChemIDplus is a free, web search system that provides access to the structure and nomenclature authority files used for the identification of chemical substances cited in National Library of Medicine (NLM) databases, including the TOXNET system. | |
Record name | (2R,3S)-1-[(4-Aminobenzene-1-sulfonyl)(2-methylpropyl)amino]-3-[({[(3R,3aS,6aR)-hexahydrofuro[2,3-b]furan-3-yl]oxy}carbonyl)amino]-4-phenylbutan-2-yl beta-D-glucopyranosiduronic acid | |
Source | EPA DSSTox | |
URL | https://comptox.epa.gov/dashboard/DTXSID50747418 | |
Description | DSSTox provides a high quality public chemistry resource for supporting improved predictive toxicology. | |
Record name | (1R,2S)-1-((((4-AMINOPHENYL)SULFONYL)(2-METHYLPROPYL)AMINO)METHYL)-2-(((((3R,3AS,6AR)-HEXAHYDROFURO(2,3-B)FURAN-3-YL)OXY)CARBONYL)AMINO)-3-PHENYLPROPYL .BETA.-D-GLUCOPYRANOSIDURONIC ACID | |
Source | FDA Global Substance Registration System (GSRS) | |
URL | https://gsrs.ncats.nih.gov/ginas/app/beta/substances/H2IG33O2AN | |
Description | The FDA Global Substance Registration System (GSRS) enables the efficient and accurate exchange of information on what substances are in regulated products. Instead of relying on names, which vary across regulatory domains, countries, and regions, the GSRS knowledge base makes it possible for substances to be defined by standardized, scientific descriptions. | |
Explanation | Unless otherwise noted, the contents of the FDA website (www.fda.gov), both text and graphics, are not copyrighted. They are in the public domain and may be republished, reprinted and otherwise used freely by anyone without the need to obtain permission from FDA. Credit to the U.S. Food and Drug Administration as the source is appreciated but not required. | |
Descargo de responsabilidad e información sobre productos de investigación in vitro
Tenga en cuenta que todos los artículos e información de productos presentados en BenchChem están destinados únicamente con fines informativos. Los productos disponibles para la compra en BenchChem están diseñados específicamente para estudios in vitro, que se realizan fuera de organismos vivos. Los estudios in vitro, derivados del término latino "in vidrio", involucran experimentos realizados en entornos de laboratorio controlados utilizando células o tejidos. Es importante tener en cuenta que estos productos no se clasifican como medicamentos y no han recibido la aprobación de la FDA para la prevención, tratamiento o cura de ninguna condición médica, dolencia o enfermedad. Debemos enfatizar que cualquier forma de introducción corporal de estos productos en humanos o animales está estrictamente prohibida por ley. Es esencial adherirse a estas pautas para garantizar el cumplimiento de los estándares legales y éticos en la investigación y experimentación.