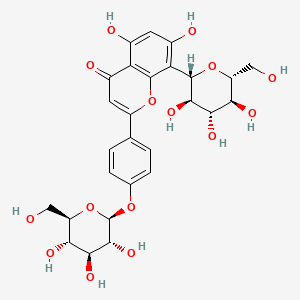
Vitexin 4'-glucósido
Descripción general
Descripción
Vitexin 4’-glucoside is a naturally occurring flavonoid glycoside found in various plants, including Briza stricta. It is known for its diverse biological activities, including anti-inflammatory, antioxidant, and neuroprotective effects . This compound is a derivative of vitexin, which is apigenin-8-C-glucoside, and it has an additional glucose moiety attached at the 4’ position.
Aplicaciones Científicas De Investigación
Vitexin 4’-glucoside has a wide range of scientific research applications:
Chemistry: Used as a model compound for studying glycosylation reactions and the synthesis of glycosides.
Biology: Investigated for its role in cellular signaling pathways and its effects on cell proliferation and apoptosis.
Medicine: Explored for its potential therapeutic effects in treating neurodegenerative diseases like Alzheimer’s and Parkinson’s due to its neuroprotective properties
Industry: Utilized in the development of functional foods and nutraceuticals due to its antioxidant and anti-inflammatory properties
Mecanismo De Acción
Target of Action
Vitexin 4’-glucoside, also known as 4’-O-Glucosylvitexin, primarily targets glutamate, a neurotransmitter that can impact brain function . It also interacts with acetylcholinesterase (AChE) and butyrylcholinesterase (BChE) . These enzymes play a crucial role in the nervous system, and their inhibition can lead to increased levels of acetylcholine, a neurotransmitter essential for memory and learning .
Mode of Action
Vitexin 4’-glucoside effectively inhibits glutamate and suppresses the catalytic role of AChE and BChE . This inhibition leads to an increase in acetylcholine levels, which can impact brain function . It also regulates the release of important inflammatory cytokines like tumor necrosis factor-Alpha (TNF-a), Interleukin 1-b (IL-1b), and Interleukin-6 (IL-6) and enzymes (iNOS, MMP-1, MMP-3, and MMP-13) .
Biochemical Pathways
Vitexin 4’-glucoside affects the nitric oxide synthase (iNOS) pathway . The activation of iNOS leads to the production of high concentrations of Nitric Oxide (NO) through the activation of inducible nuclear factors, including nuclear factor kappa B (NF-κB) . Vitexin transforms to the aglycone structure and the glucose moiety is cleaved by the bacteria. The aglycone structure was then degraded to small phenolic acids .
Pharmacokinetics
After intravenous administration, Vitexin 4’-glucoside fits a two-compartment open model . The values of AUC increased proportionally within the range of 20–60 mg/kg . The terminal half-life of Vitexin 4’-glucoside in rats showed significant differences between 20 mg/kg and other doses . This suggests that Vitexin 4’-glucoside presents a dose-dependent pharmacokinetics in the range of 20–60 mg/kg and non-linear pharmacokinetics at lower dose .
Result of Action
Vitexin 4’-glucoside exhibits a wide range of biological activities, including anti-inflammatory, antioxidant, and neuroprotective effects . It has demonstrated the ability to suppress the production of pro-inflammatory cytokines and mitigate oxidative stress .
Action Environment
The action of Vitexin 4’-glucoside is influenced by environmental factors such as pH. Vitexin-loaded chitosan nanoparticles are stable in acidic and neutral pH media, and the loaded vitexin can potentially withstand the harsh gastrointestinal (GI) environment . The in vitro simulated GI digestion confirms the enhanced bioaccessibility of vitexin after loading into chitosan nanoparticles .
Análisis Bioquímico
Biochemical Properties
Vitexin 4’-glucoside interacts with various enzymes and proteins in biochemical reactions. It effectively inhibits glutamate, a neurotransmitter that can impact brain function . Additionally, it has demonstrated the ability to suppress the production of pro-inflammatory cytokines and mitigate oxidative stress .
Cellular Effects
Vitexin 4’-glucoside has significant effects on various types of cells and cellular processes. It has been shown to protect ECV304 cells from peroxidation induced by tertbutyl hydroperoxide . It also exhibits anti-tumor activities, as demonstrated in human breast cancer cytotoxicity assays .
Molecular Mechanism
At the molecular level, Vitexin 4’-glucoside exerts its effects through various mechanisms. It has been suggested that it acts mainly by the modulation of GABAergic neurotransmission and/or related pathways . This could explain its dual activity as an anticonvulsant and anxiolytic.
Temporal Effects in Laboratory Settings
In laboratory settings, the effects of Vitexin 4’-glucoside change over time. It has been found that Vitexin 4’-glucoside-loaded chitosan nanoparticles are stable in acidic and neutral pH media, and the loaded vitexin can potentially withstand the harsh gastrointestinal environment .
Dosage Effects in Animal Models
In animal models, the effects of Vitexin 4’-glucoside vary with different dosages. For instance, it has been shown that the systemic administration of Vitexin 4’-glucoside exhibited selective protection against chemically-induced seizures .
Metabolic Pathways
Vitexin 4’-glucoside is involved in various metabolic pathways. It transforms to the aglycone structure and the glucose moiety is cleaved by bacteria. The aglycone structure is then degraded to small phenolic acids including 3-(4-hydroxyphenyl) propionic acid, phenylacetic .
Transport and Distribution
It has been suggested that Vitexin 4’-glucoside-loaded chitosan nanoparticles improve its dispersion stability in water .
Métodos De Preparación
Synthetic Routes and Reaction Conditions: Vitexin 4’-glucoside can be synthesized enzymatically using glycosyltransferases and glycosidases. Glycosyltransferases catalyze the formation of glycosidic bonds using activated sugar donors like uridine-diphosphate glucose. Glycosidases, on the other hand, operate under thermodynamic control using non-activated sugar donors, making them cost-effective for glycoside synthesis .
Industrial Production Methods: The industrial production of vitexin 4’-glucoside involves the use of solvent-stable β-fructosidase in organic solvents. This enzyme shows high activity and stability in 30–80% ethyl acetate, achieving yields of 90–99% for vitexin glycosides. The process involves the use of 50% ethyl acetate solvent system to convert vitexin into vitexin 4’-glucoside .
Análisis De Reacciones Químicas
Types of Reactions: Vitexin 4’-glucoside undergoes various chemical reactions, including glycosylation, oxidation, and reduction. Glycosylation is particularly significant as it enhances the solubility and bioactivity of the compound .
Common Reagents and Conditions:
Glycosylation: Utilizes glycosyltransferases or glycosidases in the presence of activated or non-activated sugar donors.
Oxidation and Reduction: Standard reagents like hydrogen peroxide for oxidation and sodium borohydride for reduction are used under mild conditions.
Major Products: The primary product of glycosylation is vitexin 4’-glucoside itself, while oxidation and reduction reactions can lead to various oxidized or reduced derivatives of the compound.
Comparación Con Compuestos Similares
Vitexin 4’-glucoside is unique due to its additional glucose moiety at the 4’ position, which enhances its solubility and bioactivity compared to vitexin. Similar compounds include:
Vitexin (apigenin-8-C-glucoside): Lacks the additional glucose moiety, resulting in lower solubility.
Isovitexin (apigenin-6-C-glucoside): Similar structure but with the glucose moiety at the 6 position, leading to different biological activities
Actividad Biológica
Vitexin 4'-glucoside (VOG) is a flavonoid glycoside derived from vitexin, a C-glucoside flavone known for its diverse biological activities. This compound has garnered attention due to its potential therapeutic applications in various health conditions, particularly in cardiovascular diseases and oxidative stress-related disorders.
Chemical Structure and Properties
VOG is characterized by the presence of a glucose molecule attached to the 4' position of the vitexin structure. Its molecular formula is , with a molecular weight of approximately 432.38 g/mol. The glycosylation enhances its solubility compared to vitexin, thus improving its bioavailability and pharmacological potential.
Antioxidant Activity
VOG has been shown to exhibit significant antioxidant properties. In a study involving human adipose-derived stem cells (hADSCs), VOG demonstrated protective effects against hydrogen peroxide-induced oxidative stress. Pretreatment with VOG at concentrations of 120 μM significantly reduced cell apoptosis and morphological damage caused by oxidative stress, as evidenced by decreased caspase-3 activity and lower rates of apoptosis/necrosis .
Table 1: Effects of VOG on hADSCs
Treatment Concentration (μM) | Apoptosis Rate (%) | Caspase-3 Activity (Relative Units) | Morphological Changes |
---|---|---|---|
Control | 30 | 100 | Normal |
VOG (120 μM) | 10 | 30 | Minimal Distortion |
H2O2 (500 μM) | 80 | 90 | Severe Distortion |
Cardiovascular Protection
VOG, along with vitexin-2"-O-rhamnoside, has been extensively studied for its cardioprotective effects. Both compounds are derived from Cratagus pinnatifida, a plant traditionally used in herbal medicine for cardiovascular health. Research indicates that VOG can mitigate oxidative stress in cardiac cells, enhancing cell survival and function under stress conditions .
The mechanisms underlying the biological activities of VOG primarily involve:
- Antioxidant Mechanism : VOG scavenges free radicals and enhances the activity of endogenous antioxidant enzymes, thereby reducing oxidative damage to cells.
- Anti-inflammatory Effects : VOG may inhibit pro-inflammatory cytokines and pathways, contributing to its protective effects in various tissues.
- Cell Survival Pathways : Activation of survival pathways such as PI3K/Akt has been suggested as a mechanism through which VOG exerts its protective effects against apoptosis.
Case Studies and Research Findings
Several studies have highlighted the efficacy of VOG in clinical and experimental settings:
- A study investigating the effect of VOG on hADSCs showed that it significantly reduces oxidative stress-induced damage, suggesting potential applications in regenerative medicine .
- Another research focused on the enzymatic synthesis of novel vitexin glucosides, including VOG, indicated that glycosylation improves solubility and bioavailability, enhancing the pharmacological profile of vitexin derivatives .
Propiedades
IUPAC Name |
5,7-dihydroxy-8-[(2S,3R,4R,5S,6R)-3,4,5-trihydroxy-6-(hydroxymethyl)oxan-2-yl]-2-[4-[(2S,3R,4R,5S,6R)-3,4,5-trihydroxy-6-(hydroxymethyl)oxan-2-yl]oxyphenyl]chromen-4-one | |
---|---|---|
Source | PubChem | |
URL | https://pubchem.ncbi.nlm.nih.gov | |
Description | Data deposited in or computed by PubChem | |
InChI |
InChI=1S/C27H30O15/c28-7-15-19(33)21(35)23(37)26(41-15)18-12(31)5-11(30)17-13(32)6-14(40-25(17)18)9-1-3-10(4-2-9)39-27-24(38)22(36)20(34)16(8-29)42-27/h1-6,15-16,19-24,26-31,33-38H,7-8H2/t15-,16-,19-,20-,21+,22+,23-,24-,26+,27-/m1/s1 | |
Source | PubChem | |
URL | https://pubchem.ncbi.nlm.nih.gov | |
Description | Data deposited in or computed by PubChem | |
InChI Key |
CQJPSSJEHVNDFL-WIQAIWCDSA-N | |
Source | PubChem | |
URL | https://pubchem.ncbi.nlm.nih.gov | |
Description | Data deposited in or computed by PubChem | |
Canonical SMILES |
C1=CC(=CC=C1C2=CC(=O)C3=C(O2)C(=C(C=C3O)O)C4C(C(C(C(O4)CO)O)O)O)OC5C(C(C(C(O5)CO)O)O)O | |
Source | PubChem | |
URL | https://pubchem.ncbi.nlm.nih.gov | |
Description | Data deposited in or computed by PubChem | |
Isomeric SMILES |
C1=CC(=CC=C1C2=CC(=O)C3=C(O2)C(=C(C=C3O)O)[C@H]4[C@@H]([C@H]([C@@H]([C@H](O4)CO)O)O)O)O[C@H]5[C@@H]([C@H]([C@@H]([C@H](O5)CO)O)O)O | |
Source | PubChem | |
URL | https://pubchem.ncbi.nlm.nih.gov | |
Description | Data deposited in or computed by PubChem | |
Molecular Formula |
C27H30O15 | |
Source | PubChem | |
URL | https://pubchem.ncbi.nlm.nih.gov | |
Description | Data deposited in or computed by PubChem | |
Molecular Weight |
594.5 g/mol | |
Source | PubChem | |
URL | https://pubchem.ncbi.nlm.nih.gov | |
Description | Data deposited in or computed by PubChem | |
Descargo de responsabilidad e información sobre productos de investigación in vitro
Tenga en cuenta que todos los artículos e información de productos presentados en BenchChem están destinados únicamente con fines informativos. Los productos disponibles para la compra en BenchChem están diseñados específicamente para estudios in vitro, que se realizan fuera de organismos vivos. Los estudios in vitro, derivados del término latino "in vidrio", involucran experimentos realizados en entornos de laboratorio controlados utilizando células o tejidos. Es importante tener en cuenta que estos productos no se clasifican como medicamentos y no han recibido la aprobación de la FDA para la prevención, tratamiento o cura de ninguna condición médica, dolencia o enfermedad. Debemos enfatizar que cualquier forma de introducción corporal de estos productos en humanos o animales está estrictamente prohibida por ley. Es esencial adherirse a estas pautas para garantizar el cumplimiento de los estándares legales y éticos en la investigación y experimentación.