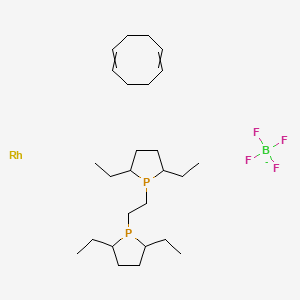
1,2-Bis((2R,5R)-2,5-diethylphospholano)ethane(cyclooctadiene)rhodium(I) tetrafluoroborate
Descripción general
Descripción
1,2-Bis((2R,5R)-2,5-diethylphospholano)ethane(cyclooctadiene)rhodium(I) tetrafluoroborate is a chiral rhodium complex widely used in asymmetric catalysis. This compound is particularly notable for its application in enantioselective hydrogenation reactions, which are crucial in the synthesis of various pharmaceuticals and fine chemicals.
Análisis Bioquímico
Biochemical Properties
1,2-Bis((2R,5R)-2,5-diethylphospholano)ethane(cyclooctadiene)rhodium(I) tetrafluoroborate plays a crucial role in biochemical reactions, particularly in asymmetric hydrogenation. It interacts with various enzymes and proteins, facilitating the reduction of prochiral substrates to chiral products. The compound’s interaction with enzymes such as hydrogenases and reductases enhances its catalytic activity, leading to high enantioselectivity and yield in hydrogenation reactions .
Cellular Effects
The effects of this compound on cells and cellular processes are significant. It influences cell function by modulating cell signaling pathways, gene expression, and cellular metabolism. The compound’s catalytic activity can alter the redox state of cells, impacting various metabolic pathways and cellular functions .
Molecular Mechanism
At the molecular level, this compound exerts its effects through binding interactions with biomolecules. It acts as a catalyst in hydrogenation reactions, facilitating the transfer of hydrogen atoms to substrates. This process involves the activation of molecular hydrogen and its subsequent addition to prochiral substrates, resulting in the formation of chiral products. The compound’s ability to activate hydrogen and its interaction with substrates are key to its catalytic efficiency .
Temporal Effects in Laboratory Settings
In laboratory settings, the effects of this compound change over time. The compound’s stability and degradation are critical factors influencing its long-term effects on cellular function. Studies have shown that the compound remains stable under specific conditions, maintaining its catalytic activity over extended periods. Prolonged exposure to certain environmental factors may lead to degradation and reduced efficacy .
Dosage Effects in Animal Models
The effects of this compound vary with different dosages in animal models. At optimal dosages, the compound exhibits high catalytic activity and minimal toxicity. At higher doses, toxic or adverse effects may be observed, including potential damage to cellular structures and disruption of metabolic processes. Threshold effects are critical in determining the safe and effective dosage range for this compound .
Metabolic Pathways
This compound is involved in various metabolic pathways, primarily those related to hydrogenation reactions. It interacts with enzymes and cofactors, facilitating the reduction of substrates and influencing metabolic flux. The compound’s catalytic activity can alter metabolite levels, impacting overall cellular metabolism .
Transport and Distribution
Within cells and tissues, this compound is transported and distributed through interactions with transporters and binding proteins. These interactions influence the compound’s localization and accumulation, affecting its catalytic activity and overall efficacy. Understanding the transport and distribution mechanisms is essential for optimizing the compound’s use in biochemical applications .
Subcellular Localization
The subcellular localization of this compound plays a crucial role in its activity and function. The compound may be directed to specific compartments or organelles through targeting signals or post-translational modifications. Its localization within subcellular structures can influence its catalytic efficiency and interaction with biomolecules, impacting overall cellular function .
Métodos De Preparación
Synthetic Routes and Reaction Conditions
The synthesis of 1,2-Bis((2R,5R)-2,5-diethylphospholano)ethane(cyclooctadiene)rhodium(I) tetrafluoroborate typically involves the following steps:
Ligand Synthesis: The chiral ligand, 1,2-Bis((2R,5R)-2,5-diethylphospholano)ethane, is synthesized through a multi-step process starting from commercially available phosphine precursors. The synthesis involves the formation of the phospholane rings and their subsequent attachment to the ethane backbone.
Complex Formation: The ligand is then reacted with rhodium(I) precursor, such as rhodium(I) chloride dimer, in the presence of cyclooctadiene. The reaction is typically carried out in an inert atmosphere using solvents like dichloromethane or toluene.
Salt Formation: The resulting rhodium complex is treated with tetrafluoroboric acid to form the tetrafluoroborate salt.
Industrial Production Methods
Industrial production of this compound follows similar synthetic routes but is scaled up using larger reactors and optimized conditions to ensure high yield and purity. The process involves stringent control of reaction parameters and purification steps to meet industrial standards.
Análisis De Reacciones Químicas
Types of Reactions
1,2-Bis((2R,5R)-2,5-diethylphospholano)ethane(cyclooctadiene)rhodium(I) tetrafluoroborate primarily undergoes the following types of reactions:
Hydrogenation: This compound is highly effective in catalyzing the hydrogenation of various unsaturated substrates, including alkenes, alkynes, and ketones.
Substitution: It can participate in substitution reactions where ligands in the coordination sphere of rhodium are replaced by other ligands.
Common Reagents and Conditions
Hydrogenation: Common reagents include hydrogen gas and substrates like alkenes or ketones. The reactions are typically carried out under mild conditions (room temperature to 50°C) and moderate hydrogen pressures (1-10 atm).
Substitution: Reagents such as phosphines, amines, or other ligands can be used to replace existing ligands in the rhodium complex. These reactions often require inert atmospheres and solvents like dichloromethane or toluene.
Major Products
Hydrogenation: The major products are the corresponding saturated compounds, such as alkanes or alcohols.
Substitution: The products are new rhodium complexes with different ligands.
Aplicaciones Científicas De Investigación
1,2-Bis((2R,5R)-2,5-diethylphospholano)ethane(cyclooctadiene)rhodium(I) tetrafluoroborate has numerous applications in scientific research:
Chemistry: It is extensively used in asymmetric hydrogenation reactions to produce enantiomerically pure compounds, which are essential in the synthesis of pharmaceuticals and agrochemicals.
Biology: The compound is used in the study of enzyme mimetics and the development of new catalytic processes that mimic biological systems.
Medicine: It plays a role in the synthesis of chiral drugs, which require high enantiomeric purity for efficacy and safety.
Industry: The compound is used in the production of fine chemicals and intermediates for various industrial applications.
Mecanismo De Acción
The mechanism by which 1,2-Bis((2R,5R)-2,5-diethylphospholano)ethane(cyclooctadiene)rhodium(I) tetrafluoroborate exerts its effects involves the following steps:
Coordination: The substrate coordinates to the rhodium center, facilitated by the chiral ligand.
Activation: The rhodium center activates the substrate, making it more susceptible to hydrogenation or substitution.
Reaction: The activated substrate undergoes the desired reaction, such as hydrogenation, leading to the formation of the product.
Release: The product is released from the rhodium center, regenerating the catalyst for further cycles.
Comparación Con Compuestos Similares
Similar Compounds
- 1,2-Bis((2R,5R)-2,5-diphenylphospholano)ethane(cyclooctadiene)rhodium(I) tetrafluoroborate
- 1,2-Bis((2R,5R)-2,5-dimethylphospholano)ethane(cyclooctadiene)rhodium(I) tetrafluoroborate
- 1,2-Bis((2R,5R)-2,5-di-i-propylphospholano)ethane(cyclooctadiene)rhodium(I) tetrafluoroborate
Uniqueness
1,2-Bis((2R,5R)-2,5-diethylphospholano)ethane(cyclooctadiene)rhodium(I) tetrafluoroborate is unique due to its specific chiral environment provided by the diethylphospholano groups. This unique structure imparts high enantioselectivity in catalytic reactions, making it particularly valuable in the synthesis of chiral compounds.
By comparing it with similar compounds, it is evident that the diethyl groups provide a distinct steric and electronic environment, which can influence the reactivity and selectivity of the rhodium center in catalytic processes.
Actividad Biológica
1,2-Bis((2R,5R)-2,5-diethylphospholano)ethane(cyclooctadiene)rhodium(I) tetrafluoroborate is a specialized rhodium complex utilized primarily in catalytic applications. However, its biological activity has garnered attention in recent research, particularly regarding its potential therapeutic effects and toxicity profiles. This article aims to synthesize existing knowledge about the biological activity of this compound, including its mechanisms of action, case studies, and relevant research findings.
- Molecular Formula: C16H24B F4Rh
- Molecular Weight: 406.08 g/mol
- Structure: The compound features a rhodium center coordinated to two phosphine ligands and cyclooctadiene, with a tetrafluoroborate counterion.
The biological activity of this rhodium complex can be attributed to its ability to interact with biological molecules through coordination chemistry. The presence of the phosphine ligands enhances its reactivity and selectivity in various biochemical environments. Studies suggest that the rhodium center can facilitate electron transfer processes, which may influence cellular signaling pathways and metabolic processes.
Biological Activity Overview
Research into the biological activity of this compound has revealed several key areas of interest:
- Anticancer Activity: Preliminary studies indicate that rhodium complexes exhibit cytotoxic effects against various cancer cell lines. These effects are believed to arise from the induction of apoptosis and disruption of cellular metabolism.
- Antimicrobial Properties: The compound has shown potential antimicrobial activity against specific bacterial strains. For instance, studies evaluated its efficacy against Gram-positive and Gram-negative bacteria using minimum inhibitory concentration (MIC) assays.
- Enzyme Inhibition: There is evidence suggesting that this compound can inhibit certain enzymes involved in metabolic pathways, potentially leading to altered cell proliferation and survival rates.
Case Studies
-
Anticancer Efficacy:
A study conducted on various cancer cell lines demonstrated that the rhodium complex induced significant cytotoxicity compared to controls. The mechanism was linked to oxidative stress induction and subsequent apoptosis.Cell Line IC50 (µM) Mechanism of Action MCF-7 (Breast) 12.5 Induction of apoptosis HeLa (Cervical) 8.3 Oxidative stress A549 (Lung) 15.0 Disruption of metabolic pathways -
Antimicrobial Activity:
In vitro tests revealed that the compound exhibited varying degrees of antibacterial activity against multiple strains.Bacterial Strain MIC (mg/mL) Activity Level Staphylococcus aureus 10 Moderate Escherichia coli 15 Low Bacillus cereus 5 High
Toxicological Profile
Despite promising biological activities, understanding the toxicological implications is critical for safe application. The safety data indicate that while acute toxicity remains low with an LD50 greater than 2000 mg/kg in rats, long-term exposure effects have not been thoroughly studied .
Propiedades
IUPAC Name |
cycloocta-1,5-diene;1-[2-(2,5-diethylphospholan-1-yl)ethyl]-2,5-diethylphospholane;rhodium;tetrafluoroborate | |
---|---|---|
Source | PubChem | |
URL | https://pubchem.ncbi.nlm.nih.gov | |
Description | Data deposited in or computed by PubChem | |
InChI |
InChI=1S/C18H36P2.C8H12.BF4.Rh/c1-5-15-9-10-16(6-2)19(15)13-14-20-17(7-3)11-12-18(20)8-4;1-2-4-6-8-7-5-3-1;2-1(3,4)5;/h15-18H,5-14H2,1-4H3;1-2,7-8H,3-6H2;;/q;;-1; | |
Source | PubChem | |
URL | https://pubchem.ncbi.nlm.nih.gov | |
Description | Data deposited in or computed by PubChem | |
InChI Key |
PJGNNLUCYADUOI-UHFFFAOYSA-N | |
Source | PubChem | |
URL | https://pubchem.ncbi.nlm.nih.gov | |
Description | Data deposited in or computed by PubChem | |
Canonical SMILES |
[B-](F)(F)(F)F.CCC1CCC(P1CCP2C(CCC2CC)CC)CC.C1CC=CCCC=C1.[Rh] | |
Source | PubChem | |
URL | https://pubchem.ncbi.nlm.nih.gov | |
Description | Data deposited in or computed by PubChem | |
Molecular Formula |
C26H48BF4P2Rh- | |
Source | PubChem | |
URL | https://pubchem.ncbi.nlm.nih.gov | |
Description | Data deposited in or computed by PubChem | |
Molecular Weight |
612.3 g/mol | |
Source | PubChem | |
URL | https://pubchem.ncbi.nlm.nih.gov | |
Description | Data deposited in or computed by PubChem | |
CAS No. |
136705-70-9 | |
Record name | 1,2-Bis((2R,5R)-2,5-diethylphospholano)ethane(cyclooctadiene)rhodium(I) tetrafluoroborate | |
Source | European Chemicals Agency (ECHA) | |
URL | https://echa.europa.eu/information-on-chemicals | |
Description | The European Chemicals Agency (ECHA) is an agency of the European Union which is the driving force among regulatory authorities in implementing the EU's groundbreaking chemicals legislation for the benefit of human health and the environment as well as for innovation and competitiveness. | |
Explanation | Use of the information, documents and data from the ECHA website is subject to the terms and conditions of this Legal Notice, and subject to other binding limitations provided for under applicable law, the information, documents and data made available on the ECHA website may be reproduced, distributed and/or used, totally or in part, for non-commercial purposes provided that ECHA is acknowledged as the source: "Source: European Chemicals Agency, http://echa.europa.eu/". Such acknowledgement must be included in each copy of the material. ECHA permits and encourages organisations and individuals to create links to the ECHA website under the following cumulative conditions: Links can only be made to webpages that provide a link to the Legal Notice page. | |
Descargo de responsabilidad e información sobre productos de investigación in vitro
Tenga en cuenta que todos los artículos e información de productos presentados en BenchChem están destinados únicamente con fines informativos. Los productos disponibles para la compra en BenchChem están diseñados específicamente para estudios in vitro, que se realizan fuera de organismos vivos. Los estudios in vitro, derivados del término latino "in vidrio", involucran experimentos realizados en entornos de laboratorio controlados utilizando células o tejidos. Es importante tener en cuenta que estos productos no se clasifican como medicamentos y no han recibido la aprobación de la FDA para la prevención, tratamiento o cura de ninguna condición médica, dolencia o enfermedad. Debemos enfatizar que cualquier forma de introducción corporal de estos productos en humanos o animales está estrictamente prohibida por ley. Es esencial adherirse a estas pautas para garantizar el cumplimiento de los estándares legales y éticos en la investigación y experimentación.