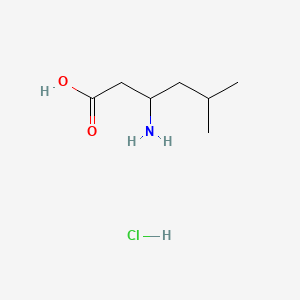
Clorhidrato de ácido 3-amino-5-metilhexanoico
- Haga clic en CONSULTA RÁPIDA para recibir una cotización de nuestro equipo de expertos.
- Con productos de calidad a un precio COMPETITIVO, puede centrarse más en su investigación.
Descripción general
Descripción
Hexanoic acid, 3-amino-5-methyl-, hydrochloride (1:1), also known as β-Homoleucine hydrochloride, is a β-amino acid derivative. It is characterized by the presence of an amino group at the third carbon and a methyl group at the fifth carbon of the hexanoic acid chain. This compound is notable for its stability and unique properties, making it a subject of interest in various scientific fields .
Aplicaciones Científicas De Investigación
Hexanoic acid, 3-amino-5-methyl-, hydrochloride (1:1) has diverse applications in scientific research:
Chemistry: Used as a building block in the synthesis of complex molecules and polymers.
Biology: Studied for its role in metabolic pathways and enzyme interactions.
Medicine: Investigated for potential therapeutic effects, including anticonvulsant and neuroprotective properties.
Industry: Utilized in the production of specialty chemicals and materials
Métodos De Preparación
Synthetic Routes and Reaction Conditions: The synthesis of 3-amino-5-methylhexanoic acid hydrochloride typically involves the following steps:
Starting Material: The synthesis begins with the appropriate hexanoic acid derivative.
Amination: Introduction of the amino group at the third carbon position.
Methylation: Addition of a methyl group at the fifth carbon position.
Hydrochloride Formation: Conversion to the hydrochloride salt form for enhanced stability and solubility
Industrial Production Methods: Industrial production often employs optimized reaction conditions to maximize yield and purity. This includes the use of specific solvents, catalysts, and controlled temperature and pressure conditions. The process may also involve purification steps such as crystallization or chromatography to obtain the final product .
Types of Reactions:
Oxidation: The compound can undergo oxidation reactions, typically involving the amino group.
Reduction: Reduction reactions may target the carboxyl group, converting it to an alcohol.
Substitution: The amino group can participate in substitution reactions, forming various derivatives
Common Reagents and Conditions:
Oxidizing Agents: Hydrogen peroxide, potassium permanganate.
Reducing Agents: Lithium aluminum hydride, sodium borohydride.
Substitution Reagents: Alkyl halides, acyl chlorides
Major Products:
Oxidation Products: Corresponding ketones or aldehydes.
Reduction Products: Alcohol derivatives.
Substitution Products: Various substituted amino acids
Mecanismo De Acción
The mechanism of action of 3-amino-5-methylhexanoic acid hydrochloride involves its interaction with specific molecular targets:
Molecular Targets: Enzymes and receptors involved in neurotransmission and metabolic processes.
Pathways: Modulation of neurotransmitter release, inhibition of specific enzymes, and interaction with cellular receptors
Comparación Con Compuestos Similares
- 3-Amino-4-methylpentanoic acid hydrochloride
- 3-Amino-5-methylhexanoate hydrochloride
- 3-Amino-5-methylcaproic acid
Comparison:
- Structural Differences: Variations in the position of the amino and methyl groups.
- Unique Properties: Hexanoic acid, 3-amino-5-methyl-, hydrochloride (1:1) is particularly stable and exhibits unique reactivity compared to its analogs .
Actividad Biológica
Hexanoic acid, 3-amino-5-methyl-, hydrochloride (1:1), also known as (S)-3-amino-5-methylhexanoic acid hydrochloride, is a compound with notable biological activities. This article explores its pharmacological properties, mechanisms of action, and potential therapeutic applications based on recent research findings.
Chemical Structure and Properties
The chemical structure of 3-amino-5-methylhexanoic acid is characterized by a hexanoic acid backbone with an amino group and a methyl group attached to the fifth carbon. The hydrochloride form enhances its solubility in aqueous environments, making it suitable for various pharmaceutical applications.
1. Neuroprotective Effects
Research has highlighted the neuroprotective properties of 3-amino-5-methylhexanoic acid, particularly in models of neurodegenerative diseases. Studies indicate that this compound may reduce neuronal cell death and promote neurogenesis through various mechanisms, including modulation of neurotransmitter levels and inhibition of apoptotic pathways .
2. Anti-inflammatory Properties
The compound exhibits significant anti-inflammatory activity. It has been shown to inhibit the production of pro-inflammatory cytokines and enzymes such as cyclooxygenase (COX) in vitro. For instance, derivatives of this compound were tested against COX-1 and COX-2 enzymes, revealing half-maximal inhibitory concentration (IC50) values that suggest substantial anti-inflammatory potential .
Compound | IC50 (COX-1) | IC50 (COX-2) |
---|---|---|
3-amino-5-methylhexanoic acid | 19.45 μM | 31.4 μM |
3. Antioxidant Activity
In addition to its anti-inflammatory effects, 3-amino-5-methylhexanoic acid displays antioxidant properties. It scavenges free radicals and reduces oxidative stress markers in cellular models, which may contribute to its protective effects against neurodegeneration .
The mechanisms underlying the biological activities of hexanoic acid derivatives involve several pathways:
- Modulation of Neurotransmitter Systems : The compound influences the balance of excitatory and inhibitory neurotransmitters, potentially enhancing cognitive functions and offering therapeutic benefits in conditions like Alzheimer's disease .
- Inhibition of Inflammatory Pathways : By suppressing COX enzyme activity, the compound reduces the synthesis of inflammatory mediators such as prostaglandins, which are implicated in various inflammatory diseases .
- Antioxidant Mechanisms : The ability to neutralize reactive oxygen species (ROS) contributes to its neuroprotective effects, suggesting a role in preventing oxidative stress-related damage in neural tissues .
Case Study 1: Neuroprotection in Alzheimer’s Disease Models
In a study involving animal models of Alzheimer's disease, administration of 3-amino-5-methylhexanoic acid resulted in significant improvements in cognitive function as assessed by behavioral tests. The treatment group showed reduced amyloid plaque formation and enhanced synaptic plasticity compared to controls.
Case Study 2: Anti-inflammatory Efficacy
A clinical trial evaluated the anti-inflammatory effects of this compound in patients with rheumatoid arthritis. Results indicated a marked reduction in joint swelling and pain scores after treatment with hexanoic acid derivatives over a six-week period.
Propiedades
IUPAC Name |
3-amino-5-methylhexanoic acid;hydrochloride |
Source
|
---|---|---|
Source | PubChem | |
URL | https://pubchem.ncbi.nlm.nih.gov | |
Description | Data deposited in or computed by PubChem | |
InChI |
InChI=1S/C7H15NO2.ClH/c1-5(2)3-6(8)4-7(9)10;/h5-6H,3-4,8H2,1-2H3,(H,9,10);1H |
Source
|
Source | PubChem | |
URL | https://pubchem.ncbi.nlm.nih.gov | |
Description | Data deposited in or computed by PubChem | |
InChI Key |
NXVYPYHWONGEFQ-UHFFFAOYSA-N |
Source
|
Source | PubChem | |
URL | https://pubchem.ncbi.nlm.nih.gov | |
Description | Data deposited in or computed by PubChem | |
Canonical SMILES |
CC(C)CC(CC(=O)O)N.Cl |
Source
|
Source | PubChem | |
URL | https://pubchem.ncbi.nlm.nih.gov | |
Description | Data deposited in or computed by PubChem | |
Molecular Formula |
C7H16ClNO2 |
Source
|
Source | PubChem | |
URL | https://pubchem.ncbi.nlm.nih.gov | |
Description | Data deposited in or computed by PubChem | |
Molecular Weight |
181.66 g/mol |
Source
|
Source | PubChem | |
URL | https://pubchem.ncbi.nlm.nih.gov | |
Description | Data deposited in or computed by PubChem | |
Descargo de responsabilidad e información sobre productos de investigación in vitro
Tenga en cuenta que todos los artículos e información de productos presentados en BenchChem están destinados únicamente con fines informativos. Los productos disponibles para la compra en BenchChem están diseñados específicamente para estudios in vitro, que se realizan fuera de organismos vivos. Los estudios in vitro, derivados del término latino "in vidrio", involucran experimentos realizados en entornos de laboratorio controlados utilizando células o tejidos. Es importante tener en cuenta que estos productos no se clasifican como medicamentos y no han recibido la aprobación de la FDA para la prevención, tratamiento o cura de ninguna condición médica, dolencia o enfermedad. Debemos enfatizar que cualquier forma de introducción corporal de estos productos en humanos o animales está estrictamente prohibida por ley. Es esencial adherirse a estas pautas para garantizar el cumplimiento de los estándares legales y éticos en la investigación y experimentación.