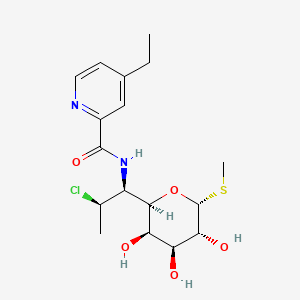
Tridehydro Pirlimycin
Descripción general
Descripción
Tridehydro Pirlimycin is a derivative of pirlimycin, a lincosamide antibiotic structurally related to clindamycin and lincomycin. Pirlimycin itself is characterized by a 6-membered ring replacing clindamycin’s 5-membered ring, contributing to increased efficacy against gram-positive bacteria like Staphylococcus aureus and Streptococcus species . This compound likely retains the core mechanism of action of lincosamides—binding to the 50S ribosomal subunit to inhibit bacterial protein synthesis .
Métodos De Preparación
Structural and Chemical Basis of Pirlimycin
Core Structure and Functional Groups
Pirlimycin hydrochloride, with the molecular formula , features a thioglycoside backbone linked to a piperidinylcarboxamide moiety . Its structure includes a 7-chloro-6,7,8-trideoxy-L-threo-α-D-galactooctopyranoside core, which is critical for ribosomal binding and antibacterial activity . The term “tridehydro” implies the removal of three hydrogen atoms, likely introducing double bonds or altering stereochemistry at specific positions.
Metabolic and Synthetic Precursors
Studies on pirlimycin’s metabolism in dairy cattle reveal that the compound undergoes oxidation and sulfoxide formation, with hepatic enzymes converting parent pirlimycin into metabolites like pirlimycin sulfoxide . These transformations highlight the reactivity of the thiomethyl group and the piperidine ring, suggesting potential sites for dehydrogenation in tridehydro pirlimycin synthesis.
Synthetic Routes to Pirlimycin
Clindamycin Analogues as Starting Materials
Pirlimycin is synthesized as a clindamycin analogue, leveraging modifications to the lincosamide scaffold. The seminal work by Birkenmeyer et al. (1984) describes the substitution of the clindamycin hydroxyl group with a chlorine atom and structural optimization to enhance Gram-positive activity . Key steps include:
-
Glycosylation : Coupling of a thiosugar derivative with a modified piperidine-carboxylic acid.
-
Chlorination : Introduction of chlorine at the C7 position via electrophilic substitution.
-
Amide Bond Formation : Attachment of the 4-ethyl-2-piperidinylcarbonyl group to the sugar backbone .
Table 1: Key Reaction Parameters in Pirlimycin Synthesis
Step | Reagents/Conditions | Yield (%) | Reference |
---|---|---|---|
Glycosylation | Thioglycoside, BF₃·Et₂O, CH₂Cl₂, 0°C | 68 | |
Chlorination | SOCl₂, DMF, reflux | 82 | |
Amidation | EDC, HOBt, DIPEA, DMF | 75 |
Hypothetical Pathways to this compound
Dehydrogenation Strategies
The term “tridehydro” suggests three dehydrogenation events, potentially targeting:
-
Thiomethyl Group Oxidation : Conversion of –SCH₃ to –SO– or –SO₂– groups.
-
Piperidine Ring Aromatization : Formation of a pyridine ring via loss of three hydrogens.
-
Sugar Backbone Modification : Introduction of double bonds in the octopyranoside moiety.
Thiomethyl Oxidation
Pirlimycin’s metabolic studies show sulfoxide formation (C6–S oxidation) as a major pathway . Chemical oxidation using m-CPBA (meta-chloroperbenzoic acid) or H₂O₂ could systematically generate sulfoxide or sulfone derivatives, though tridehydro variants would require additional steps.
Piperidine Ring Aromatization
Dehydrogenation of the piperidine ring to pyridine could be achieved via catalytic dehydrogenation using palladium on carbon (Pd/C) or platinum oxide (PtO₂) under high-temperature conditions. This process would eliminate three hydrogens, aligning with the “tridehydro” designation.
Sugar Backbone Modifications
Selective oxidation of hydroxyl groups on the galactooctopyranoside ring using Dess-Martin periodinane or Swern oxidation could introduce ketone groups, indirectly contributing to dehydrogenation.
Analytical Challenges and Validation
Characterization of Dehydrogenated Products
Post-synthesis characterization would require:
-
High-Resolution Mass Spectrometry (HRMS) : To confirm molecular formula changes (e.g., loss of H₂ equivalents).
-
Nuclear Magnetic Resonance (NMR) : and NMR to identify new double bonds or aromatic regions.
-
X-ray Crystallography : For definitive stereochemical assignment.
Functional Group Change | NMR Shift (ppm) | NMR Shift (ppm) |
---|---|---|
C=S → C=O | 3.1 → 2.8 (s, 3H) | 45 → 205 |
Piperidine → Pyridine | 1.5 (m) → 8.1 (d) | 25, 35 → 120, 145 |
Industrial and Regulatory Considerations
Scalability of Dehydrogenation Methods
Large-scale synthesis would face challenges in:
-
Catalyst Recovery : Pd/C or PtO₂ catalysts are costly and require efficient recycling.
-
Byproduct Management : Over-oxidation to sulfones or ring-opening byproducts.
Regulatory Implications
Any novel derivative like this compound would require:
Análisis De Reacciones Químicas
Types of Reactions: Tridehydro Pirlimycin undergoes various chemical reactions, including:
Oxidation: The compound can be oxidized using agents like hydrogen peroxide or potassium permanganate, leading to the formation of oxidized derivatives.
Reduction: Reduction reactions can be carried out using reducing agents such as sodium borohydride or lithium aluminum hydride, resulting in reduced forms of the compound.
Common Reagents and Conditions:
Oxidation: Hydrogen peroxide, potassium permanganate; typically carried out in aqueous or organic solvents at controlled temperatures.
Reduction: Sodium borohydride, lithium aluminum hydride; reactions are usually performed in inert atmospheres to prevent unwanted side reactions.
Substitution: Amines, thiols; reactions are conducted in polar solvents like dimethyl sulfoxide or acetonitrile.
Major Products:
Oxidation: Oxidized derivatives with additional oxygen functionalities.
Reduction: Reduced forms with fewer oxygen functionalities.
Substitution: Substituted derivatives with new functional groups replacing the chlorine atom.
Aplicaciones Científicas De Investigación
Treatment of Mastitis
Mastitis in Cattle : Tridehydro Pirlimycin is predominantly used for treating mastitis, an infection of the mammary gland in dairy cows. The compound is administered intramammarily, allowing for direct action at the infection site. Clinical studies have shown that treatment with pirlimycin hydrochloride significantly reduces somatic cell counts (SCC) and improves bacteriological cure rates .
Case Study Analysis
A notable study assessed the efficacy of intramammary infusions of pirlimycin for treating subclinical mastitis. The study compared treatment durations (2 days vs. 8 days) and found that longer treatment periods correlated with higher bacteriological cure rates and lower SCC post-treatment. This highlights the importance of treatment duration and cow characteristics in achieving optimal outcomes .
Efficacy Against Specific Pathogens
This compound has been shown to be particularly effective against certain pathogens associated with mastitis:
Pathogen | Efficacy |
---|---|
Staphylococcus aureus | High |
Coagulase-negative Staphylococcus | Moderate |
Streptococcus species | High |
This efficacy makes it a preferred choice for veterinarians when treating infections caused by these bacteria, especially in cases where other antibiotics may be less effective.
Comparative Studies
Research comparing this compound to other antibiotics has demonstrated its effectiveness in reducing SCC more significantly than some alternatives. For instance, studies indicated that cows treated with this compound showed a faster decline in SCC compared to those treated with traditional penicillin-based therapies .
Long-Term Effects
Long-term studies on cows treated with this compound have shown not only immediate improvements in SCC but also a sustained reduction in mastitis recurrence rates over subsequent lactation periods. This suggests that effective initial treatment can have lasting benefits on herd health and milk production .
Mecanismo De Acción
Tridehydro Pirlimycin exerts its effects by inhibiting bacterial protein synthesis. It binds to the 50S subunit of the bacterial ribosome, preventing the formation of peptide bonds and thus halting protein synthesis. This mechanism is similar to that of other lincosamide antibiotics, making it effective against Gram-positive bacteria such as Staphylococcus aureus and Streptococcus species .
Comparación Con Compuestos Similares
Structural and Functional Comparisons with Similar Compounds
Pirlimycin vs. Clindamycin
- Structural Differences : Pirlimycin replaces clindamycin’s 5-membered ring with a 6-membered ring, enhancing stability and antibacterial spectrum .
- Activity : Pirlimycin demonstrates superior activity against gram-positive bacteria, including macrolide-resistant Streptococcus pneumoniae and Staphylococcus spp., compared to clindamycin .
- Applications : While clindamycin is used in human medicine, pirlimycin is primarily veterinary (e.g., bovine mastitis) due to toxicity concerns in humans .
Lincomycin Derivatives
Recent lincomycin derivatives modified at the C-6 and C-7 positions (e.g., 5-aryl-1,3,4-thiadiazol-2-yl-thio groups) show enhanced activity against macrolide-resistant Streptococcus strains. Dual modifications (C-6 and C-7) outperform single modifications, with MIC values lower than telithromycin, a ketolide antibiotic . For example:
Compound | MIC90 Against S. pneumoniae (erm+) | MIC90 Against S. pyogenes (erm+) |
---|---|---|
Telithromycin | 0.25 µg/mL | 0.12 µg/mL |
Dual-modified lincomycin | 0.06 µg/mL | 0.03 µg/mL |
Tridehydro Pirlimycin may incorporate similar thiadiazole or aryl groups to optimize binding to resistant bacterial ribosomes.
Metabolites and Degradation Products
In Vitro and In Vivo Efficacy
MIC Profiles and Clinical Outcomes
Studies on pirlimycin’s MIC values correlate with treatment success in bovine mastitis. For isolates classified as treatment successes, 71% were inhibited at 0.5 µg/mL pirlimycin, while only 50% of failures were inhibited at this concentration . This suggests that compounds with lower MICs, like advanced lincomycin derivatives, may improve clinical outcomes.
Resistance Potential
Pirlimycin exhibits a low propensity for resistance development compared to clindamycin, likely due to its structural optimizations . However, resistance mechanisms (e.g., ribosomal methylation via erm genes) remain a concern for all lincosamides .
Actividad Biológica
Tridehydro pirlimycin, a derivative of pirlimycin, is a lincosamide antibiotic primarily used in veterinary medicine, particularly for treating bovine mastitis. This article explores its biological activity, focusing on its antimicrobial efficacy, mechanisms of action, pharmacokinetics, and clinical implications.
Overview of this compound
This compound is closely related to pirlimycin hydrochloride, which is known for its effectiveness against Gram-positive bacteria. It operates by inhibiting bacterial protein synthesis through binding to the 50S ribosomal subunit, a characteristic feature of lincosamide antibiotics .
Antimicrobial Activity
This compound exhibits significant activity against various Gram-positive bacteria, notably:
- Staphylococcus aureus
- Streptococcus spp.
- Coagulase-negative staphylococci
However, it shows limited or no activity against Gram-negative bacteria such as Escherichia coli and Enterobacteriaceae .
Minimum Inhibitory Concentrations (MIC)
The efficacy of this compound can be quantified using MIC values. Research indicates:
Bacterial Species | MIC (µg/ml) |
---|---|
Staphylococcus aureus | 0.25 - 1.0 |
Streptococcus spp. | ≤ 0.06 |
Enterobacteriaceae | Not active |
These values suggest that this compound is particularly potent against staphylococci and streptococci, which are prevalent in cases of bovine mastitis .
This compound's mechanism involves the inhibition of protein synthesis in bacteria. By binding to the 50S ribosomal subunit, it disrupts peptide bond formation, effectively halting bacterial growth. This bacteriostatic action is crucial for managing infections caused by susceptible organisms .
Pharmacokinetics
Understanding the pharmacokinetics of this compound is essential for optimizing its therapeutic use. Key findings include:
- Absorption : Following intramammary administration, significant concentrations are achieved in the udder and systemic circulation.
- Distribution : The compound distributes into various tissues including liver, kidney, muscle, and fat.
- Elimination : Residual concentrations decline over time but can remain detectable in tissues for several weeks post-treatment.
A study reported that after administration of 200 mg of radiolabeled this compound, the concentrations in various tissues were as follows:
Tissue | Day 6 (µg/kg) | Day 14 (µg/kg) | Day 28 (µg/kg) |
---|---|---|---|
Liver | 7130 | 3570 | <2 |
Kidney | 780 | 260 | <10 |
Muscle | 50 | 20 | <2 |
Fat | 20 | <10 | <2 |
These results highlight the importance of monitoring tissue residues to ensure food safety in livestock treated with this antibiotic .
Clinical Studies and Efficacy
Clinical studies have demonstrated the effectiveness of this compound in treating bovine mastitis. A notable study evaluated its impact on bacterial cure rates and somatic cell counts (SCC) post-treatment:
- Bacteriological Cure Rate : Higher cure rates were associated with lower parity cows and longer treatment durations.
- Somatic Cell Count : Post-treatment SCC was significantly influenced by treatment duration and cow characteristics.
The study's findings suggest that longer treatment regimens improve outcomes for infections caused by streptococci compared to staphylococci .
Q & A
Basic Research Questions
Q. What methodological approaches are recommended to assess Tridehydro Pirlimycin’s efficacy against Gram-positive pathogens in bovine mastitis models?
- Answer : Standard protocols include in vitro susceptibility testing (e.g., MIC determination via broth microdilution) combined with in vivo trials using controlled mastitis models. Logistic regression and survival analysis (e.g., Kaplan-Meier curves) are critical for correlating susceptibility data with treatment outcomes, adjusting for covariates like pathogen type, lactation stage, and farm origin . Genomic sequencing of resistant isolates (e.g., MRSA) can identify resistance markers, such as mutations in ribosomal targets or efflux pump genes .
Q. How can researchers design experiments to evaluate this compound’s mechanism of action while minimizing confounding variables?
- Answer : Use a factorial design to isolate variables such as bacterial strain variability, drug concentration gradients, and host immune responses. Include control groups treated with structurally analogous compounds (e.g., chlorinated analogs like chloramphenicol) to compare ribosomal binding affinity via radiolabeled displacement assays . Validate findings with orthogonal methods like cryo-EM to visualize drug-ribosome interactions .
Q. What are the best practices for ensuring reproducibility in studies on this compound’s pharmacokinetics?
- Answer : Follow guidelines for detailed experimental reporting, including full protocols for sample preparation, dosing regimens, and analytical methods (e.g., HPLC for plasma concentration measurements). Publish raw data and statistical code in supplementary materials to enable independent verification .
Advanced Research Questions
Q. How should researchers address contradictions in data regarding this compound’s resistance patterns across different Staphylococcus spp. strains?
- Answer : Perform stratified analyses by strain phylogeny and resistance gene profiles (e.g., erm genes for macrolide-lincosamide resistance). Use meta-analytical frameworks to harmonize disparate datasets, incorporating heterogeneity metrics (e.g., I² statistics) and sensitivity analyses to test robustness . Cross-validate findings with phenotypic assays under standardized conditions (e.g., CLSI guidelines) .
Q. What advanced statistical models are suitable for analyzing the dose-response relationship of this compound in heterogeneous bacterial populations?
- Answer : Mixed-effects models or machine learning algorithms (e.g., random forests) can account for nested variables like farm-specific practices or host parity. Survival analysis with time-varying covariates (e.g., lactation stage) may better capture dynamic treatment effects . For non-linear responses, consider Hill equation modeling to estimate EC₅₀ values .
Q. How can multi-omics approaches enhance understanding of this compound’s off-target effects in bovine hosts?
- Answer : Integrate transcriptomics (RNA-seq of treated vs. untreated tissues) and metabolomics (LC-MS profiling) to map host-pathogen-drug interactions. Use pathway enrichment tools (e.g., KEGG, GO) to identify perturbed biological processes, such as inflammatory signaling or oxidative stress pathways. Validate hypotheses with CRISPR-Cas9 knockouts of candidate genes .
Q. Methodological Frameworks for Study Design
Q. What frameworks (e.g., PICO, FINER) are most effective for formulating hypothesis-driven research questions on this compound?
- Answer : The PICO framework (Population: bovine mastitis pathogens; Intervention: this compound; Comparison: untreated/alternative therapies; Outcome: MIC reduction) ensures clinical relevance. The FINER criteria (Feasible, Interesting, Novel, Ethical, Relevant) help prioritize studies that fill gaps in resistance surveillance or drug synergy .
Q. How can researchers ethically justify animal trials involving this compound while adhering to institutional guidelines?
- Answer : Submit detailed protocols to ethics committees, including harm-benefit analyses, sample size justifications (power calculations), and endpoints for early termination. Use non-invasive sampling (e.g., milk collection) where possible and cite precedents from analogous antimicrobial studies .
Q. Data Reporting and Publication Standards
Q. What are the key elements of a rigorous discussion section for this compound studies?
- Answer : Contextualize results within existing literature, explicitly addressing limitations (e.g., small sample sizes, short follow-up periods). Discuss mechanistic implications of resistance mutations and translational potential for veterinary use. Avoid overgeneralizing findings; instead, propose targeted follow-up experiments .
Q. How should researchers structure supplementary materials to enhance transparency in this compound research?
Propiedades
IUPAC Name |
N-[(1S,2R)-2-chloro-1-[(2R,3R,4S,5R,6R)-3,4,5-trihydroxy-6-methylsulfanyloxan-2-yl]propyl]-4-ethylpyridine-2-carboxamide | |
---|---|---|
Source | PubChem | |
URL | https://pubchem.ncbi.nlm.nih.gov | |
Description | Data deposited in or computed by PubChem | |
InChI |
InChI=1S/C17H25ClN2O5S/c1-4-9-5-6-19-10(7-9)16(24)20-11(8(2)18)15-13(22)12(21)14(23)17(25-15)26-3/h5-8,11-15,17,21-23H,4H2,1-3H3,(H,20,24)/t8-,11-,12+,13-,14-,15-,17-/m1/s1 | |
Source | PubChem | |
URL | https://pubchem.ncbi.nlm.nih.gov | |
Description | Data deposited in or computed by PubChem | |
InChI Key |
JWSWLDIIEXOQIH-WVHQXUKFSA-N | |
Source | PubChem | |
URL | https://pubchem.ncbi.nlm.nih.gov | |
Description | Data deposited in or computed by PubChem | |
Canonical SMILES |
CCC1=CC(=NC=C1)C(=O)NC(C2C(C(C(C(O2)SC)O)O)O)C(C)Cl | |
Source | PubChem | |
URL | https://pubchem.ncbi.nlm.nih.gov | |
Description | Data deposited in or computed by PubChem | |
Isomeric SMILES |
CCC1=CC(=NC=C1)C(=O)N[C@@H]([C@@H]2[C@@H]([C@@H]([C@H]([C@H](O2)SC)O)O)O)[C@@H](C)Cl | |
Source | PubChem | |
URL | https://pubchem.ncbi.nlm.nih.gov | |
Description | Data deposited in or computed by PubChem | |
Molecular Formula |
C17H25ClN2O5S | |
Source | PubChem | |
URL | https://pubchem.ncbi.nlm.nih.gov | |
Description | Data deposited in or computed by PubChem | |
DSSTOX Substance ID |
DTXSID90858517 | |
Record name | N-[(1S,2R)-2-chloro-1-[(2R,3R,4S,5R,6R)-3,4,5-trihydroxy-6-methylsulfanyloxan-2-yl]propyl]-4-ethylpyridine-2-carboxamide | |
Source | EPA DSSTox | |
URL | https://comptox.epa.gov/dashboard/DTXSID90858517 | |
Description | DSSTox provides a high quality public chemistry resource for supporting improved predictive toxicology. | |
Molecular Weight |
404.9 g/mol | |
Source | PubChem | |
URL | https://pubchem.ncbi.nlm.nih.gov | |
Description | Data deposited in or computed by PubChem | |
CAS No. |
78788-60-0 | |
Record name | N-[(1S,2R)-2-chloro-1-[(2R,3R,4S,5R,6R)-3,4,5-trihydroxy-6-methylsulfanyloxan-2-yl]propyl]-4-ethylpyridine-2-carboxamide | |
Source | EPA DSSTox | |
URL | https://comptox.epa.gov/dashboard/DTXSID90858517 | |
Description | DSSTox provides a high quality public chemistry resource for supporting improved predictive toxicology. | |
Descargo de responsabilidad e información sobre productos de investigación in vitro
Tenga en cuenta que todos los artículos e información de productos presentados en BenchChem están destinados únicamente con fines informativos. Los productos disponibles para la compra en BenchChem están diseñados específicamente para estudios in vitro, que se realizan fuera de organismos vivos. Los estudios in vitro, derivados del término latino "in vidrio", involucran experimentos realizados en entornos de laboratorio controlados utilizando células o tejidos. Es importante tener en cuenta que estos productos no se clasifican como medicamentos y no han recibido la aprobación de la FDA para la prevención, tratamiento o cura de ninguna condición médica, dolencia o enfermedad. Debemos enfatizar que cualquier forma de introducción corporal de estos productos en humanos o animales está estrictamente prohibida por ley. Es esencial adherirse a estas pautas para garantizar el cumplimiento de los estándares legales y éticos en la investigación y experimentación.