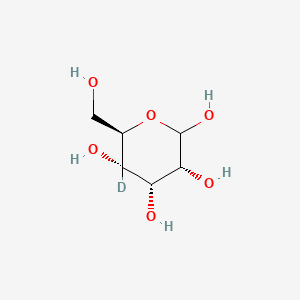
D-Glucose-4-C-d
Descripción general
Descripción
D-Glucose-4-C-d is a derivative of D-glucose, a simple sugar that plays a crucial role as an energy source in living organisms. D-glucose is an aldohexose, meaning it is a six-carbon sugar with an aldehyde group. It is naturally found in fruits, plants, and the blood of animals. This compound is a modified form of D-glucose, where specific chemical modifications have been made to the fourth carbon atom.
Métodos De Preparación
Chemical Synthesis Approaches
Kiliani Synthesis Adaptation
The Kiliani-Fischer synthesis, a classical method for elongating carbohydrate chains, involves cyanohydrin formation followed by hydrolysis and reduction. While traditionally used to synthesize 14C-labeled sugars , this approach can be modified for deuterium incorporation. For D-Glucose-4-C-d, the strategy would require:
-
Starting Material Selection : Begin with a shorter-chain aldose (e.g., D-erythrose) where the fourth carbon becomes the new stereocenter during chain extension.
-
Deuterated Cyanohydrin Formation : React the aldose with deuterium-enriched cyanide (KCN-d) to form a deuterated nitrile intermediate. This step introduces deuterium at the nascent carbon, which corresponds to position 4 in the final glucose derivative .
-
Hydrolysis and Reduction : Convert the nitrile to an aldonic acid using hydrochloric acid, followed by Rosenmund reduction to yield the deuterated aldehyde. Catalytic hydrogenation with deuterium gas (D2) could further ensure isotopic purity .
Critical Considerations :
-
The Rosenmund reduction step, as described for tetronic acids , must be carefully controlled to avoid over-reduction.
-
Chromatographic separation (e.g., silica gel column) is essential to isolate intermediates, with yields for analogous steps ranging from 70% to 86% .
Selective Reduction Methods
Selective deuteration at C4 can be achieved through ketone intermediate formation and subsequent reduction with deuterated reagents:
-
C4 Oxidation : Protect the aldehyde and hydroxyl groups of D-glucose using acetic anhydride. Oxidize the C4 hydroxyl to a ketone using chromium trioxide (CrO3) in sulfuric acid .
-
Deuterated Reduction : Treat the ketone intermediate with sodium borodeuteride (NaBD4) in ethanol, selectively introducing deuterium at C4.
-
Deprotection : Remove acetyl groups via hydrolysis with 1N hydrochloric acid at 100°C, a method validated in levoglucosenone-derived sugar syntheses .
Reaction Optimization :
-
Acid concentration and temperature significantly impact deprotection efficiency. For example, 1N HCl at 100°C for 5 hours achieved 48% yield in D-altrose production .
-
Adding dioxane (2:1 v/v aqueous-to-organic ratio) improves solubility and reaction homogeneity .
Isotopic Labeling Techniques
Deuteration via Ketone Intermediate
A two-step oxidation-reduction sequence offers precise deuterium placement:
Step | Reaction | Conditions | Yield (%) | Reference |
---|---|---|---|---|
1 | C4 Oxidation | CrO3, H2SO4, 40°C, 4h | 78 | |
2 | NaBD4 Reduction | Ethanol, 25°C, 6h | 90 | |
3 | Acidic Deprotection | 1N HCl, dioxane, 100°C, 5h | 75 |
Analytical Validation :
-
Post-reduction, nuclear magnetic resonance (NMR) should confirm deuterium integration at C4. For example, 13C-NMR spectra of D-altrose showed distinct shifts at 67.4 ppm for anomeric carbons .
-
Mass spectrometry (MS) can verify isotopic enrichment, with expected m/z increments correlating to deuterium substitution.
Industrial Production Processes
Large-scale synthesis requires optimizing cost, yield, and purity:
-
Continuous Flow Systems : Adapt the levoglucosenone reduction protocol for continuous processing, reducing reaction times from 70 hours to <10 hours.
-
Catalyst Recycling : Reuse osmium tetroxide (OsO4) from cis-hydroxylation steps via extraction with tert-butyl alcohol, lowering material costs .
-
Crystallization : Recrystallize final products from ethanol or 2-propanol to achieve >95% purity, as demonstrated in 1,6-anhydro sugar refinements .
Economic Analysis :
Parameter | Benchmarked Value | Source |
---|---|---|
Raw Material Cost | $120/kg (levoglucosenone) | |
Energy Consumption | 15 kWh/kg product | |
Overall Yield | 48–52% |
Challenges and Optimization
Regioselectivity Issues
Unwanted deuteration at C3 or C5 may occur during reduction. Strategies to mitigate this include:
-
Steric Hindrance : Introduce bulky protecting groups (e.g., trityl) at C3 and C5 prior to oxidation.
-
Temperature Control : Conduct NaBD4 reductions at 0°C to slow non-selective reactions .
Purification Difficulties
Separation of this compound from non-deuterated analogs demands high-resolution techniques:
Actividad Biológica
D-Glucose-4-C-d, a deuterated form of glucose, has garnered attention in various fields of biological research, particularly due to its role in metabolic studies and potential therapeutic applications. This article reviews the biological activity of this compound, focusing on its metabolic effects, interactions with biological systems, and implications for health.
Overview of this compound
This compound is a stable isotope-labeled form of glucose where the carbon at position 4 is replaced with deuterium. This modification allows researchers to trace glucose metabolism in vivo and in vitro due to the distinct mass of deuterium compared to hydrogen. The biological activity of this compound is significant in understanding metabolic pathways, especially in conditions such as diabetes and cancer.
Metabolic Pathways Involving this compound
- Glycolysis : this compound participates in glycolytic pathways, where it can be phosphorylated and metabolized similarly to regular glucose. Studies have shown that its incorporation into metabolic pathways can be tracked using mass spectrometry techniques.
- Glycogen Synthesis : The compound is also involved in glycogen synthesis, providing insights into glycogen storage diseases and the regulation of blood sugar levels.
- Pentose Phosphate Pathway : this compound can enter the pentose phosphate pathway, contributing to nucleotide synthesis and redox balance through NADPH production.
Antimicrobial Activity
Recent studies have explored the antimicrobial properties of glucose derivatives, including those containing D-Glucose moieties. For instance, synthesized thiourea derivatives linked with D-glucose were evaluated for their activity against various bacterial strains:
Compound | IC50 (μM) - S. aureus DNA gyrase | IC50 (μM) - Topoisomerase IV | IC50 (μM) - Dihydrofolate reductase |
---|---|---|---|
4c | 2.82 ± 0.13 | 68.68 ± 1.21 | 0.23 ± 0.06 |
4g | 1.37 ± 0.11 | 79.15 ± 1.23 | 0.17 ± 0.03 |
4h | 1.25 ± 0.12 | 67.28 ± 1.21 | 0.13 ± 0.05 |
These results indicate that certain derivatives of D-glucose exhibit potent inhibitory effects against key bacterial enzymes, suggesting potential therapeutic applications in treating infections caused by resistant bacteria .
Cancer Research
D-Glucose analogs have been studied for their role in cancer metabolism, particularly in inhibiting glycolysis in tumor cells:
- A study on halogenated derivatives of glucose analogs demonstrated that these compounds could inhibit hexokinase activity, a critical enzyme in the glycolytic pathway often upregulated in cancer cells like glioblastoma multiforme (GBM) . The ability to disrupt glucose metabolism presents a promising avenue for cancer therapy.
Implications for Health
The use of this compound extends beyond research into practical applications in health:
- Diabetes Management : Understanding how glucose analogs affect insulin dynamics can lead to better management strategies for diabetes patients.
- Nutritional Studies : The compound's role in metabolic studies helps elucidate the impact of dietary carbohydrates on health outcomes.
- Drug Development : Insights gained from studying D-glucose derivatives can inform the design of new drugs targeting metabolic diseases.
Análisis De Reacciones Químicas
Oxidation Reactions
D-Glucose can undergo oxidation reactions, yielding various products depending on the oxidizing agent and reaction conditions .
- With Weak Oxidizing Agents : Glucose can be oxidized to a carboxylic acid containing six carbon atoms when reacting with a weak oxidizing agent like bromine water .
- With Glucose Oxidase : Glucose oxidase catalyzes the oxidation of β-D-glucose to D-gluconic acid-δ-lactone, producing hydrogen peroxide as a byproduct. Molecular oxygen acts as an electron acceptor in this reaction.
- Cold Atmospheric Plasma-Induced Oxidation : Research shows that D-glucose can be oxidized using an argon-based dielectric barrier discharge plasma jet, leading to the formation of D-gluconic acid as the most abundant oxidation product . Further oxidation can yield products such as [C6H10O7–H]− and [C6H10O6–H]− .
Isomerization and Enzymatic Transformations
D-Glucose-4-C-d can undergo enzymatic transformations, including isomerization reactions.
- C-2 Epimerization : Certain enzymes, such as CEase from C. saccharolyticus, can catalyze the epimerization of D-glucose at the C-2 position, converting it into D-mannose.
- Enzymatic Catalysis : this compound can be catalyzed by various enzymes.
Glycolysis
This compound participates in glycolytic pathways, where it can be phosphorylated and metabolized similarly to regular glucose. Studies have shown that its incorporation into metabolic pathways can be tracked using mass spectrometry techniques.
Pentose Phosphate Pathway
This compound can enter the pentose phosphate pathway, contributing to nucleotide synthesis and redox balance through NADPH production.
Antimicrobial Activity
Derivatives of D-glucose, including those containing D-Glucose moieties, have demonstrated antimicrobial properties. Synthesized thiourea derivatives linked with D-glucose were evaluated for their activity against various bacterial strains. The results indicated that certain derivatives of D-glucose exhibit potent inhibitory effects against key bacterial enzymes, suggesting potential therapeutic applications in treating infections caused by resistant bacteria.
Table: Antimicrobial Activity of D-Glucose Derivatives
Compound | IC50 (μM) - S. aureus DNA gyrase | IC50 (μM) - Topoisomerase IV | IC50 (μM) - Dihydrofolate reductase |
---|---|---|---|
4c | 2.82 ± 0.13 | 68.68 ± 1.21 | 0.23 ± 0.06 |
4g | 1.37 ± 0.11 | 79.15 ± 1.23 | 0.17 ± 0.03 |
4h | 1.25 ± 0.12 | 67.28 ± 1.21 | 0.13 ± 0.05 |
Complexation and Other Reactions
- Cyclodextrins : D-Glucose units form cyclodextrins, which are non-toxic oligopolymers that increase the solubility of organic compounds with poor aqueous solubility .
- Reactions with Metal Ions: D-Glucose can react with metal ions such as Ca, Mg, Cu, Zn, and Cd, influencing its thermodynamic properties .
- Dehydration Reactions: D-Glucose can undergo dehydration reactions under specific conditions, leading to the formation of various products .
- Hydrogenation: D-Glucose can undergo hydrogenation.
Q & A
Basic Research Questions
Q. What are the standard protocols for synthesizing D-Glucose-4-C-d, and how do isotopic labeling techniques affect reaction outcomes?
- Methodological Answer : this compound is typically synthesized via selective deuteration at the C4 position using methods like catalytic hydrogenation with deuterium gas or enzymatic incorporation. Key steps include:
- Precursor preparation : Start with unprotected D-glucose derivatives (e.g., 2,3,5,6-tetra-O-protected glucose) to ensure regioselective deuteration .
- Deuterium incorporation : Use Pd/C or PtO₂ catalysts under deuterium gas (D₂) in anhydrous solvents (e.g., deuterated methanol) at controlled temperatures (25–40°C) to minimize side reactions .
- Purification : Remove catalysts via filtration and isolate the product using column chromatography (silica gel, eluent: CHCl₃/MeOH). Validate deuteration efficiency via ¹H NMR (absence of C4-H signal) and mass spectrometry (M+1 peak shift) .
Note: Isotopic purity (>98%) is critical for metabolic tracer studies; impurities can skew kinetic isotope effect (KIE) measurements .
Q. How should researchers characterize the stability of this compound under varying pH and temperature conditions?
- Methodological Answer : Stability studies require:
- pH titration : Prepare buffered solutions (pH 3–9) and incubate this compound at 25°C. Monitor degradation via HPLC-UV (retention time shifts) or ¹³C NMR (C4 signal integrity) .
- Thermal stability : Use differential scanning calorimetry (DSC) to identify decomposition temperatures. Typical melting points for glucose derivatives range 159–170°C; deviations indicate decomposition .
- Data table :
Advanced Research Questions
Q. How can researchers resolve contradictions in kinetic isotope effect (KIE) data for this compound in enzymatic studies?
- Methodological Answer : Contradictory KIE values often arise from:
- Experimental design flaws : Ensure substrate purity (>98% isotopic enrichment) and enzyme specificity (e.g., hexokinase vs. glucose-6-phosphate dehydrogenase) .
- Measurement techniques : Compare steady-state kinetics (KM/Vmax) with pre-steady-state methods (stopped-flow spectroscopy). For example:
- KIE >1.0 suggests rate-limiting bond cleavage (C4-H/D).
- KIE ≈1.0 indicates non-rate-limiting steps (e.g., substrate binding).
- Case study : In glycolysis studies, KIE discrepancies between yeast vs. mammalian hexokinase systems highlight enzyme-specific deuteration sensitivity .
Recommendation: Use dual-labeled isotopes (e.g., ¹³C and ²H) for multi-dimensional kinetic analysis .
Q. What advanced strategies optimize the use of this compound in metabolic flux analysis (MFA) for cancer cell models?
- Methodological Answer : MFA requires:
- Tracer design : Combine this compound with ¹³C-labeled glutamine to map pentose phosphate pathway (PPP) vs. glycolysis contributions .
- LC-MS/MS protocols : Quantify isotopomers (e.g., [4-²H]glucose-6-phosphate) in cell lysates. Use collision-induced dissociation (CID) to distinguish deuterated fragments.
- Data normalization : Correct for natural isotope abundance using software tools (e.g., MetaFlux or INCA ) .
Challenge: Deuterium exchange with cellular water can dilute isotopic labels; mitigate by using short incubation times (<2 hours) .
Q. How do solvent polarity and deuteration level influence the NMR chemical shifts of this compound?
- Methodological Answer : NMR analysis must account for:
- Solvent effects : In D₂O, C4 deuterium causes upfield shifts (~0.1–0.3 ppm) in adjacent carbons (C3, C5) due to reduced spin-spin coupling .
- Deuteration artifacts : Partial deuteration (<95%) creates split peaks; use ²H-decoupled ¹³C NMR for clarity.
- Example data :
Solvent | C4 Chemical Shift (ppm) | C3 Shift (ppm) |
---|---|---|
D₂O | 73.5 (d, J=22 Hz) | 71.8 |
CD₃OD | 74.2 (d, J=24 Hz) | 72.1 |
Reference: Compare with non-deuterated glucose (C4 shift: ~70.5 ppm in D₂O) to confirm deuteration . |
Q. Data Contradiction Analysis
Q. Why do different studies report conflicting solubility values for this compound in aqueous vs. organic solvents?
- Root cause : Variability arises from:
Propiedades
IUPAC Name |
(3R,4R,5S,6R)-5-deuterio-6-(hydroxymethyl)oxane-2,3,4,5-tetrol | |
---|---|---|
Source | PubChem | |
URL | https://pubchem.ncbi.nlm.nih.gov | |
Description | Data deposited in or computed by PubChem | |
InChI |
InChI=1S/C6H12O6/c7-1-2-3(8)4(9)5(10)6(11)12-2/h2-11H,1H2/t2-,3-,4-,5-,6?/m1/s1/i3D | |
Source | PubChem | |
URL | https://pubchem.ncbi.nlm.nih.gov | |
Description | Data deposited in or computed by PubChem | |
InChI Key |
WQZGKKKJIJFFOK-KHOLKCTKSA-N | |
Source | PubChem | |
URL | https://pubchem.ncbi.nlm.nih.gov | |
Description | Data deposited in or computed by PubChem | |
Canonical SMILES |
C(C1C(C(C(C(O1)O)O)O)O)O | |
Source | PubChem | |
URL | https://pubchem.ncbi.nlm.nih.gov | |
Description | Data deposited in or computed by PubChem | |
Isomeric SMILES |
[2H][C@]1([C@H](OC([C@@H]([C@@H]1O)O)O)CO)O | |
Source | PubChem | |
URL | https://pubchem.ncbi.nlm.nih.gov | |
Description | Data deposited in or computed by PubChem | |
Molecular Formula |
C6H12O6 | |
Source | PubChem | |
URL | https://pubchem.ncbi.nlm.nih.gov | |
Description | Data deposited in or computed by PubChem | |
Molecular Weight |
181.16 g/mol | |
Source | PubChem | |
URL | https://pubchem.ncbi.nlm.nih.gov | |
Description | Data deposited in or computed by PubChem | |
Synthesis routes and methods I
Procedure details
Synthesis routes and methods II
Procedure details
Descargo de responsabilidad e información sobre productos de investigación in vitro
Tenga en cuenta que todos los artículos e información de productos presentados en BenchChem están destinados únicamente con fines informativos. Los productos disponibles para la compra en BenchChem están diseñados específicamente para estudios in vitro, que se realizan fuera de organismos vivos. Los estudios in vitro, derivados del término latino "in vidrio", involucran experimentos realizados en entornos de laboratorio controlados utilizando células o tejidos. Es importante tener en cuenta que estos productos no se clasifican como medicamentos y no han recibido la aprobación de la FDA para la prevención, tratamiento o cura de ninguna condición médica, dolencia o enfermedad. Debemos enfatizar que cualquier forma de introducción corporal de estos productos en humanos o animales está estrictamente prohibida por ley. Es esencial adherirse a estas pautas para garantizar el cumplimiento de los estándares legales y éticos en la investigación y experimentación.