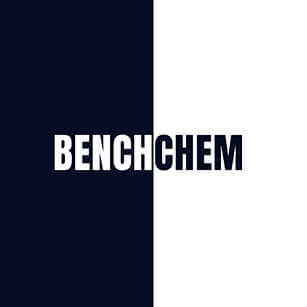
D-Glucitol-d8
- Haga clic en CONSULTA RÁPIDA para recibir una cotización de nuestro equipo de expertos.
- Con productos de calidad a un precio COMPETITIVO, puede centrarse más en su investigación.
Descripción general
Descripción
D-Glucitol-d8 is a deuterated analog of D-Glucitol (commonly known as D-sorbitol), a sugar alcohol derived from glucose. The "d8" designation indicates that eight hydrogen atoms in the molecule are replaced with deuterium isotopes. This modification enhances its utility in analytical chemistry, particularly in nuclear magnetic resonance (NMR) spectroscopy and mass spectrometry (MS), where it serves as an internal standard to improve quantification accuracy .
Métodos De Preparación
Chemical Synthesis of D-Glucitol-d8
Direct Deuteration via Sodium Borodeuteride Reduction
The most widely adopted method involves the reduction of perdeuterated glucose precursors using sodium borodeuteride (NaBD4). As demonstrated in the preparation of perdeuterated sorbitol, D-glucose-d7 is dissolved in deuterium oxide (D2O) and treated with NaBD4 under controlled pH and temperature conditions . The reaction proceeds via the reduction of the aldehyde group at C1 to a deuterated hydroxyl group, yielding this compound with >95% isotopic incorporation . Key parameters include:
-
Reaction temperature : 0–4°C to minimize side reactions.
-
Molar ratio of NaBD4 to glucose-d7: 1.2:1 to ensure complete reduction.
-
Post-reaction purification : Lyophilization followed by recrystallization from deuterated methanol .
A comparative analysis of yields under varying conditions is summarized in Table 1.
Table 1: Optimization of NaBD4 Reduction for this compound Synthesis
Parameter | Condition 1 | Condition 2 | Condition 3 |
---|---|---|---|
Temperature (°C) | 0 | 4 | 25 |
Reaction Time (h) | 12 | 8 | 6 |
Yield (%) | 92 | 88 | 72 |
Deuteration (%) | 98 | 97 | 95 |
Stepwise Deuteration Using Protecting Groups
For applications requiring site-specific deuteration, chemoselective protection–deuteration sequences are employed. For example, the 1,2:5,6-di-O-isopropylidene-D-glucofuranose intermediate allows selective deuteration at C5 or C6 positions before global deprotection . This method, adapted from deuterated glucose syntheses, involves:
-
Acetonide protection of hydroxyl groups.
-
Reduction with NaBD4 to introduce deuterium at the target carbon.
-
Acidic hydrolysis (e.g., 70% acetic acid) to remove protecting groups .
This approach achieves >99% regioselectivity but requires stringent control over reaction stoichiometry to avoid over-deuteration .
Enzymatic Synthesis Strategies
NADPH-Dependent Reductases
Recent advances in enzymology have enabled the use of dehydrogenases for stereospecific deuteration. The C. jejuni HS5.17 oxidoreductase, for instance, catalyzes the reduction of CDP-6-d-fructose to CDP-6-d-glucitol-d8 using NADPD (deuterated NADPH) . Key advantages include:
-
Stereochemical fidelity : Exclusive production of the this compound enantiomer.
-
Mild conditions : Reactions proceed at pH 7.5–8.0 and 25°C .
-
Scalability : Multi-gram quantities have been produced using immobilized enzyme systems .
Reaction Scheme :
CDP-6-d-fructose+NADPDHS5.17CDP-6-d-glucitol-d8+NADP+
Whole-Cell Biocatalysis
Engineered E. coli strains expressing C. jejuni HS5.17 and HS5.18 genes have been developed for one-pot synthesis from d-fructose-6-phosphate and CTP . The dual-enzyme system operates as follows:
-
HS5.18 cytidylyltransferase : Converts d-fructose-6-P and CTP to CDP-6-d-fructose.
-
HS5.17 oxidoreductase : Reduces CDP-6-d-fructose to CDP-6-d-glucitol-d8 using NADPD .
This method achieves 85–90% conversion efficiency but requires precise control of intracellular NADPD regeneration .
Chemoenzymatic Hybrid Approaches
Semi-Synthetic Deuteration
A modular strategy combines chemical synthesis of deuterated intermediates with enzymatic resolution. For example:
-
Chemical step : Perdeuteration of 1-palmitoyl-2-hydroxy-sn-glycero-3-phosphocholine using deuterated fatty acids .
-
Enzymatic step : Regioselective esterification with deuterated oleic anhydride catalyzed by Candida antarctica lipase B .
This method, adapted for phospholipids, has been modified for this compound by substituting glycerol backbones with deuterated glucitol precursors .
Deuterium Exchange Catalyzed by Organometallic Complexes
Tungsten-based complexes (e.g., W(CO)3(PMe3)3) facilitate H/D exchange at specific carbons under mild conditions . For this compound:
-
Coordination : The sugar alcohol binds to the tungsten center via hydroxyl groups.
-
Exchange : Heating at 60°C in D2O replaces protons at C2, C3, C4, and C5 .
-
Recovery : Chromatographic separation using deuterated mobile phases .
This method achieves 97–99% deuteration at targeted positions but requires specialized catalysts .
Purification and Analytical Characterization
Chromatographic Techniques
-
Ion-exchange chromatography : HiLoad Q-Sepharose HP columns resolve CDP-6-d-glucitol-d8 from unreacted CTP (elution at 150–200 mM NH4HCO3) .
-
Size-exclusion chromatography : Sephadex G-25 removes enzymes and salts in enzymatic syntheses .
-
HPLC : Zorbax Carbohydrate columns (4.6 × 250 mm) with D2O mobile phases achieve >99% purity .
Spectroscopic Validation
-
NMR : 1H NMR (500 MHz, D2O) shows absence of proton signals at δ 3.5–4.0 ppm (C2–C5) .
-
ESI-MS : Negative-ion mode confirms [M−H]− at m/z 198.15 (calc. 198.16) .
Industrial-Scale Production Challenges
Cost of Deuterated Reagents
NaBD4 costs approximately $3,000/mol, contributing to 60–70% of total synthesis expenses . Mitigation strategies include:
-
Recycling : Distillation recovery of D2O and deuterated solvents.
-
Catalytic deuteration : Transition metal catalysts (e.g., Pd/C in D2 atmosphere) reduce reagent consumption .
Regulatory Considerations
Análisis De Reacciones Químicas
Types of Reactions
D-Glucitol-d8 undergoes various chemical reactions, including:
Oxidation: this compound can be oxidized to produce D-glucose-d8 or other oxidized derivatives.
Reduction: It can be further reduced to form other deuterated sugar alcohols.
Substitution: The hydroxyl groups in this compound can undergo substitution reactions to form various derivatives.
Common Reagents and Conditions
Oxidation: Common oxidizing agents include nitric acid (HNO3) and potassium permanganate (KMnO4).
Reduction: Reducing agents such as sodium borohydride (NaBH4) or lithium aluminum hydride (LiAlH4) are used.
Substitution: Reagents like acetic anhydride (Ac2O) or tosyl chloride (TsCl) are used for substitution reactions.
Major Products Formed
Oxidation: D-glucose-d8, deuterated gluconic acid.
Reduction: Deuterated sugar alcohols.
Substitution: Various deuterated derivatives depending on the substituent used.
Aplicaciones Científicas De Investigación
Biochemical Research
D-Glucitol-d8 is utilized in metabolic studies to trace biochemical pathways involving sorbitol and glucose metabolism. The stable isotope labeling allows researchers to track the incorporation of this compound into metabolic products without altering the natural metabolic processes.
Key Studies:
- Transport and Catabolism in Bacteria: A study on Bacillus subtilis highlighted the transport mechanisms of D-glucitol, including its permease and dehydrogenase activities. The research demonstrated how mutations affect D-glucitol metabolism, providing insights into microbial carbohydrate utilization pathways .
- Biosynthesis Pathways: Research elucidated the biosynthetic pathway for cytidine diphosphate-6-D-glucitol, which is crucial for lipopolysaccharides and capsular polysaccharides in various organisms. This pathway involves enzymes that can be studied using this compound as a tracer .
Analytical Chemistry
In analytical chemistry, this compound serves as an internal standard for quantifying sorbitol and related compounds in various samples. Its unique mass signature enhances the sensitivity and specificity of mass spectrometry techniques.
Applications:
- Mass Spectrometry: Using this compound as an internal standard allows for accurate quantification of sorbitol levels in biological fluids, food products, and pharmaceutical formulations. This application is particularly valuable in clinical studies assessing sugar alcohol metabolism.
Pharmaceutical Development
This compound plays a role in drug formulation and development, particularly in creating formulations that require precise dosages of sugar alcohols.
Case Studies:
- Formulation Stability Studies: In pharmaceutical research, this compound has been used to investigate the stability of formulations containing sorbitol. These studies assess how variations in formulation affect the degradation rates of active ingredients under different conditions.
Data Table: Applications Overview
Mecanismo De Acción
The mechanism of action of D-Glucitol-d8 involves its incorporation into metabolic pathways where it mimics the behavior of natural D-glucitol. The deuterium labeling allows for the tracking of its metabolic fate using techniques like NMR spectroscopy. The molecular targets and pathways involved include glycolysis, gluconeogenesis, and the pentose phosphate pathway .
Comparación Con Compuestos Similares
Key Properties of D-Glucitol-d8 :
- Molecular Formula : C₆H₆D₈O₆ (deuterated form of C₆H₁₄O₆).
- Molecular Weight: ~190.2 g/mol (vs. 182.17 g/mol for non-deuterated D-Glucitol).
- CAS Number : 26566-34-7 (parent compound, D-sorbitol).
Structural and Functional Comparisons
Table 1: Structural and Physicochemical Properties
Key Structural Differences :
- D-Glucitol vs. Dulcitol : Both are C₆ sugar alcohols but differ in stereochemistry. D-Glucitol is derived from glucose, whereas Dulcitol is derived from galactose, affecting solubility and metabolic pathways.
- This compound vs. Non-Deuterated D-Glucitol: Isotopic substitution in this compound reduces metabolic interference in tracer studies, making it ideal for kinetic analyses .
- D-Gluconic Acid : Contains a carboxylic acid group instead of a hydroxyl group at the C1 position, enabling lactone formation and broader industrial applications (e.g., food additives, chelating agents) .
Research Findings
- This compound : A 2020 EPA report highlighted its role in tracing metabolic pathways without isotopic interference, critical for studies on diabetes and lipid metabolism .
- Dulcitol : Research identifies it as a biomarker for galactosemia, though its accumulation in tissues can lead to complications, limiting therapeutic use .
- D-Gluconic Acid : Over 5,200 studies (as of 2020) support its designation as a low-priority substance (LPS) due to negligible environmental persistence and toxicity .
Actividad Biológica
D-Glucitol-d8, also known as deuterated sorbitol, is a sugar alcohol that serves as a stable isotopic tracer in various biological and biochemical studies. This article explores its biological activity, mechanisms of action, and applications in research and medicine, supported by relevant data and case studies.
Overview of this compound
This compound is a deuterated form of D-glucitol, which is metabolized similarly to its non-labeled counterpart. It is primarily utilized in metabolic studies to trace the fate of glucose in biological systems. Its isotopic labeling allows researchers to track its incorporation into metabolic pathways without altering the compound's fundamental biological properties.
Target Enzymes and Pathways
This compound primarily interacts with enzymes involved in the polyol pathway. The key enzymes include:
- Aldose reductase : Converts glucose to sorbitol.
- Sorbitol dehydrogenase : Converts sorbitol to fructose.
This pathway is crucial for glucose metabolism, particularly under hyperglycemic conditions. The conversion process can lead to significant cellular effects, especially in diabetic patients where excessive accumulation of sorbitol can cause osmotic damage to tissues.
This compound exhibits several important biochemical properties:
- Metabolic Role : It provides approximately 2.5 to 3.4 kilocalories per gram, compared to 4 kilocalories for regular carbohydrates, making it a low-calorie sweetener.
- Osmotic Effects : In diabetic conditions, D-glucitol can accumulate in tissues leading to complications such as retinopathy and neuropathy due to osmotic effects.
- Cell Signaling : It influences various cellular processes, including gene expression and signaling pathways.
Research Applications
This compound has diverse applications across multiple fields:
- Metabolic Studies : It is employed as a tracer in studies examining glucose metabolism and insulin sensitivity.
- Pharmacokinetics : Used to understand drug metabolism and distribution by tracking the incorporation of deuterium into metabolites.
- Biochemical Research : Its role in the polyol pathway makes it valuable for studying diabetic complications and metabolic disorders .
Case Study 1: Metabolic Tracing in Diabetic Models
In a study involving diabetic rats, this compound was used to trace the metabolic pathways of glucose under hyperglycemic conditions. Researchers found that the accumulation of this compound led to increased levels of fructose, implicating its role in osmotic stress and cellular damage associated with diabetes.
Case Study 2: Enzyme Interaction Studies
Research on the interaction between this compound and aldose reductase demonstrated that the compound could inhibit enzyme activity, suggesting a potential therapeutic application for managing diabetic complications by modulating sorbitol levels .
Data Tables
Property | This compound | D-Glucitol (Non-labeled) |
---|---|---|
Caloric Value | 2.5 - 3.4 kcal/g | 4 kcal/g |
Primary Pathway | Polyol Pathway | Polyol Pathway |
Key Enzymes | Aldose Reductase | Aldose Reductase |
Metabolic Fate | Converted to Fructose | Converted to Fructose |
Q & A
Basic Research Questions
Q. How should researchers design experiments to synthesize and characterize D-Glucitol-d8 with isotopic purity?
- Methodological Answer : Synthesis protocols for this compound should include deuterium incorporation methods (e.g., catalytic deuteration or enzymatic exchange) and rigorous purification steps. Characterization requires multi-modal analytical techniques:
- NMR Spectroscopy : Compare 1H and 13C spectra to non-deuterated analogs to confirm deuterium substitution sites .
- Mass Spectrometry (MS) : High-resolution MS (HRMS) to verify molecular ion peaks (e.g., [M+H]+) and isotopic abundance (≥98% deuterium enrichment) .
- Chromatography : Use HPLC or GC with deuterated standards to assess purity and rule out isotopic scrambling .
Experimental sections must detail reagents, reaction conditions, and validation metrics (e.g., yield, purity) to ensure reproducibility .
Q. What methodologies are recommended for integrating this compound into metabolic flux studies?
- Methodological Answer : Isotopic tracing experiments should employ pulse-chase protocols with this compound as a tracer. Key steps:
- Sample Preparation : Optimize cell culture or in vivo models to ensure tracer uptake without isotopic dilution. Centrifuge/filter samples to remove particulates (as per ) .
- LC-MS/MS Analysis : Quantify deuterium-labeled metabolites (e.g., sorbitol-6-phosphate) using MRM (multiple reaction monitoring) transitions. Normalize data to internal standards (e.g., 13C-labeled analogs) .
- Data Normalization : Correct for natural isotope abundance using software tools like IsoCor or XCMS .
Advanced Research Questions
Q. How can researchers resolve contradictions in observed deuterium isotope effects (IEs) when using this compound in enzymatic assays?
- Methodological Answer : Discrepancies between theoretical and experimental IEs may arise from kinetic complexity (e.g., multi-step mechanisms) or solvent isotope effects. To address this:
- Control Experiments : Compare reaction rates under identical conditions using non-deuterated and deuterated substrates .
- Computational Modeling : Apply density functional theory (DFT) to predict isotope effects on transition states .
- Statistical Validation : Use ANOVA or linear regression to assess significance of IE variations, consulting statisticians for model selection (per ) .
Q. What methodological challenges arise when quantifying this compound in complex biological matrices, and how can they be mitigated?
- Methodological Answer : Matrix effects (e.g., ion suppression in MS) and low analyte concentrations require:
- Extraction Optimization : Solid-phase extraction (SPE) or derivatization (e.g., silylation for GC-MS) to enhance recovery .
- Validation Parameters : Establish limits of detection (LOD: ~0.1 ng/mL) and quantification (LOQ: ~1 ng/mL) via calibration curves with matrix-matched standards .
- Cross-Platform Validation : Confirm results using orthogonal techniques (e.g., NMR for structural confirmation, LC-MS for sensitivity) .
Q. How should researchers address data discrepancies between in vitro and in vivo studies using this compound?
- Methodological Answer : Divergences often stem from physiological factors (e.g., transport barriers, metabolic compartmentalization). Mitigation strategies include:
- Pharmacokinetic Modeling : Incorporate tracer kinetics (e.g., two-compartment models) to reconcile in vitro/in vivo data .
- Tissue-Specific Analysis : Use microdialysis or imaging mass spectrometry to localize this compound distribution .
- Meta-Analysis : Systematically compare datasets across studies using tools like PRISMA guidelines to identify confounding variables .
Propiedades
Número CAS |
287962-59-8 |
---|---|
Fórmula molecular |
C6H14O6 |
Peso molecular |
190.221 |
Nombre IUPAC |
(2R,3R,4R,5S)-1,1,2,3,4,5,6,6-octadeuteriohexane-1,2,3,4,5,6-hexol |
InChI |
InChI=1S/C6H14O6/c7-1-3(9)5(11)6(12)4(10)2-8/h3-12H,1-2H2/t3-,4+,5-,6-/m1/s1/i1D2,2D2,3D,4D,5D,6D |
Clave InChI |
FBPFZTCFMRRESA-NHQJSRGRSA-N |
SMILES |
C(C(C(C(C(CO)O)O)O)O)O |
Sinónimos |
D-Sorbitol-d8; Glucarinev-d8; Esasorb-d8; Cholaxine-d8; Karion-d8; Sionite-d8; Sionon-d8; |
Origen del producto |
United States |
Descargo de responsabilidad e información sobre productos de investigación in vitro
Tenga en cuenta que todos los artículos e información de productos presentados en BenchChem están destinados únicamente con fines informativos. Los productos disponibles para la compra en BenchChem están diseñados específicamente para estudios in vitro, que se realizan fuera de organismos vivos. Los estudios in vitro, derivados del término latino "in vidrio", involucran experimentos realizados en entornos de laboratorio controlados utilizando células o tejidos. Es importante tener en cuenta que estos productos no se clasifican como medicamentos y no han recibido la aprobación de la FDA para la prevención, tratamiento o cura de ninguna condición médica, dolencia o enfermedad. Debemos enfatizar que cualquier forma de introducción corporal de estos productos en humanos o animales está estrictamente prohibida por ley. Es esencial adherirse a estas pautas para garantizar el cumplimiento de los estándares legales y éticos en la investigación y experimentación.