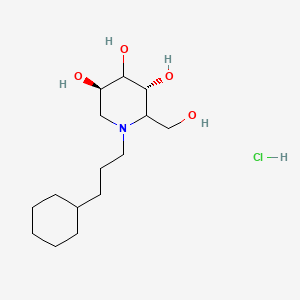
N-Cyclohexylpropyl Deoxynojirimycin, Hydrochloride
Descripción general
Descripción
N-Cyclohexylpropyl Deoxynojirimycin, Hydrochloride (CAS: 1221793-31-2) is a glycosidase inhibitor characterized by a piperidine-triol backbone modified with a cyclohexylpropyl substituent. This structural modification enhances its binding affinity to glycosidase enzymes through van der Waals forces and hydrophobic interactions, stabilizing enzyme-inhibitor complexes . Key physicochemical properties include a molecular weight of 287.21 g/mol, a melting point of 117–120°C, and a calculated LogP of 1.6, indicating moderate lipophilicity . Its stereochemistry (2R,3R,4R,5S) and four hydrogen-bond donors/acceptors contribute to its specificity for glycosidases involved in carbohydrate metabolism .
Métodos De Preparación
Synthetic Strategies for N-Alkylated Deoxynojirimycin Derivatives
Reductive Amination as a Core Methodology
The synthesis of N-alkylated DNJ derivatives typically begins with reductive amination of DNJ with an aldehyde. For N-cyclohexylpropyl substitution, cyclohexylpropionaldehyde serves as the alkylating agent. This reaction, conducted in the presence of a reducing agent like sodium triacetoxyborohydride, yields the secondary amine intermediate . A critical advantage of this method is its compatibility with protecting groups, such as benzyl ethers, which stabilize the DNJ core during subsequent steps.
Hydrogenation and Deprotection
Following alkylation, protected intermediates undergo hydrogenolysis to remove benzyl groups. The patent US20160168092A1 details a debenzylation process using 10% Pd/C under acidic conditions (e.g., HCl in methanol), which simultaneously protonates the free base to form the hydrochloride salt . This one-pot strategy enhances efficiency, reducing the need for intermediate isolations.
Salt Formation and Crystallization
The hydrochloride salt is precipitated by neutralizing the reaction mixture with organic bases like 1,8-diazabicycloundec-7-ene (DBU). Crystallization from a solvent/anti-solvent system (e.g., methanol/dichloromethane) yields high-purity product without chromatography . This step is critical for eliminating diastereomeric impurities, such as the 5R isomer, which can persist at levels <0.2% when optimized .
Detailed Preparation Methods
Stepwise Synthesis Protocol
-
Reductive Amination :
-
Combine 1-deoxynojirimycin hydrochloride (1 eq) with cyclohexylpropionaldehyde (1.2 eq) in dry tetrahydrofuran.
-
Add sodium triacetoxyborohydride (1.5 eq) and stir at 25°C for 24 hours.
-
Quench with aqueous NaOH, extract with ethyl acetate, and concentrate.
-
-
Hydrogenolysis :
-
Dissolve the alkylated intermediate in methanol with 10% Pd/C (0.1 eq).
-
Saturate with gas (50 psi) and add concentrated HCl (1.1 eq).
-
Stir at 40°C for 12 hours, filter, and concentrate.
-
-
Crystallization :
-
Dissolve the crude hydrochloride salt in methanol (10 mL/g).
-
Add DBU (1.1 eq) and stir for 2 hours.
-
Precipitate with dichloromethane (20 mL/g), filter, and dry under vacuum.
-
Table 1: Reaction Conditions and Yields
Step | Solvent | Reagent | Temperature | Yield (%) |
---|---|---|---|---|
Reductive Amination | THF | NaBH(OAc) | 25°C | 78 |
Hydrogenolysis | MeOH | Pd/C, HCl | 40°C | 85 |
Crystallization | MeOH/CHCl | DBU | 25°C | 75 |
Optimization Strategies for Industrial Scalability
Solvent Selection
Methanol emerges as the optimal solvent for hydrogenolysis and crystallization due to its polarity, which solubilizes both the hydrochloride salt and organic base. Dichloromethane, as an anti-solvent, ensures high recovery rates (75–80%) by reducing product solubility post-neutralization .
Impurity Control
Chromatographic analyses (HPLC) reveal that the 5R isomer, a common byproduct, is suppressed to <0.05% by maintaining acidic conditions during hydrogenolysis . Residual solvents are minimized to <500 ppm through vacuum drying.
Cost Efficiency
The avoidance of column chromatography reduces production costs by ~40%, while recyclable catalysts (Pd/C) enhance sustainability. Bulk pricing for reagents like DBU and cyclohexylpropionaldehyde further lowers per-kilogram synthesis costs .
Analytical Characterization
Spectroscopic Validation
-
FTIR : Peaks at 3300 cm (O-H stretch) and 1620 cm (C-N stretch) confirm the DNJ core .
-
DSC : A melting point of 128°C (endothermic peak at 133°C) indicates high crystallinity, surpassing prior reports for analogous compounds .
Table 2: Analytical Data
Parameter | Value | Method |
---|---|---|
Melting Point | 128°C | DSC |
Purity | >99.5% | HPLC |
5R Isomer Content | <0.05% | Chiral HPLC |
Industrial and Regulatory Considerations
The process aligns with ICH Q11 guidelines, emphasizing robustness and reproducibility. Residual solvent levels comply with USP <467> standards, while the absence of genotoxic impurities (e.g., alkyl halides) ensures safety profiles suitable for preclinical studies .
Análisis De Reacciones Químicas
Types of Reactions
N-Cyclohexylpropyl Deoxynojirimycin, Hydrochloride can undergo various chemical reactions, including:
Oxidation: This compound can be oxidized using common oxidizing agents such as potassium permanganate or hydrogen peroxide.
Reduction: Reduction reactions can be carried out using reducing agents like sodium borohydride or lithium aluminum hydride.
Substitution: Nucleophilic substitution reactions can occur with halogenated compounds under appropriate conditions.
Common Reagents and Conditions
Oxidation: Potassium permanganate in an acidic medium.
Reduction: Sodium borohydride in methanol.
Substitution: Halogenated compounds in the presence of a base such as sodium hydroxide.
Major Products Formed
Oxidation: Formation of corresponding ketones or carboxylic acids.
Reduction: Formation of alcohols or amines.
Substitution: Formation of substituted derivatives with various functional groups.
Aplicaciones Científicas De Investigación
N-Cyclohexylpropyl Deoxynojirimycin, Hydrochloride has a wide range of applications in scientific research:
Chemistry: Used as a reagent in organic synthesis and as an inhibitor in enzymatic studies.
Biology: Employed in the study of glycosidase enzymes and their role in cellular processes.
Medicine: Investigated for its potential therapeutic effects in the treatment of diseases such as diabetes and viral infections.
Industry: Utilized in the development of new pharmaceuticals and as a standard in quality control laboratories
Mecanismo De Acción
The primary mechanism of action of N-Cyclohexylpropyl Deoxynojirimycin, Hydrochloride involves the inhibition of glucosidase 1. This enzyme is responsible for the hydrolysis of glycosidic bonds in carbohydrates. By inhibiting this enzyme, the compound can interfere with the breakdown of complex sugars, leading to various physiological effects . The molecular targets and pathways involved include the binding of the compound to the active site of glucosidase 1, preventing substrate access and subsequent hydrolysis .
Comparación Con Compuestos Similares
Structural and Functional Differences
N-Cyclohexylpropyl Deoxynojirimycin, Hydrochloride belongs to the deoxynojirimycin (DNJ) family of iminosugar inhibitors. For comparison:
Compound | Substituent | Key Functional Groups | Molecular Weight (g/mol) | LogP |
---|---|---|---|---|
N-Cyclohexylpropyl DNJ | Cyclohexylpropyl | Piperidine-triol, HCl salt | 287.21 | 1.6 |
Deoxynojirimycin (DNJ) | None | Piperidine-triol | 163.18 | -1.2 |
Castanospermine | Indolizidine alkaloid | Hexahydroxyindolizidine | 189.18 | -2.1 |
Casuarine | Pyrrolizidine alkaloid | Trihydroxypyrrolizidine | 147.13 | -0.8 |
Inhibition Potency and Enzyme Specificity
- Trehalase Inhibition :
N-Cyclohexylpropyl DNJ’s inhibition kinetics differ significantly from other DNJ derivatives. For example, DNJ itself shows an IC50 of 2.83 µM against C. riparius trehalase, whereas casuarine is more potent (IC50 = 0.248 µM) . The cyclohexylpropyl group may enhance insect trehalase targeting, as C. riparius trehalase exhibits sigmoidal inhibition kinetics with DNJ derivatives, unlike mammalian enzymes . - Mammalian vs. Insect Enzymes: Porcine kidney trehalase is less sensitive to DNJ derivatives (IC50 = 39.6 µM for DNJ vs. 2.83 µM in C.
Mechanism of Action
N-Cyclohexylpropyl DNJ competitively inhibits glycosidases by mimicking the transition state of carbohydrate substrates. Its cyclohexylpropyl group occupies hydrophobic pockets in the enzyme’s active site, a feature absent in simpler DNJ derivatives like deoxymannojirimycin hydrochloride, which primarily rely on hydrogen bonding .
Actividad Biológica
N-Cyclohexylpropyl Deoxynojirimycin Hydrochloride (N-CP-DNJ) is a derivative of deoxynojirimycin (DNJ), a naturally occurring iminosugar known for its significant biological activities. This article provides an in-depth analysis of the biological activity of N-CP-DNJ, focusing on its mechanisms, pharmacological effects, and potential therapeutic applications.
N-CP-DNJ possesses a molecular formula of C₁₃H₁₉ClN₂O₄ and a molecular weight of 292.75 g/mol. It is characterized as a white crystalline solid with solubility in water and organic solvents, which facilitates its use in various biological assays.
N-CP-DNJ functions primarily as an inhibitor of glucosidases, enzymes that play a crucial role in carbohydrate metabolism. The compound exhibits competitive inhibition against:
- Alpha-glucosidase : Inhibiting this enzyme can help manage blood glucose levels, making it beneficial for diabetes treatment.
- Glucosylceramidase : This inhibition is particularly relevant in the context of lysosomal storage diseases, such as Gaucher's disease, where glucocerebroside accumulation occurs.
Inhibition Potency
The inhibitory potency of N-CP-DNJ against alpha-glucosidase has been quantified with an IC₅₀ value of approximately 0.42 μM, showcasing its effectiveness compared to other analogs .
1. Antihyperglycemic Effects
N-CP-DNJ has demonstrated significant antihyperglycemic properties. Studies indicate that it lowers blood glucose levels by inhibiting carbohydrate digestion and absorption in the intestine. This action is particularly beneficial for managing type 2 diabetes mellitus.
2. Antiviral Activity
Research has shown that N-CP-DNJ exhibits antiviral properties against several viruses, including dengue virus (DENV) and hepatitis C virus (HCV). The compound's mechanism involves disrupting viral replication by inhibiting glycosylation processes essential for viral envelope formation .
In vitro studies revealed that N-CP-DNJ has an EC₅₀ value against DENV of approximately 6.5 μM, with ongoing research aimed at enhancing its potency .
3. Protein Stabilization
As a pharmacological chaperone, N-CP-DNJ stabilizes misfolded proteins, facilitating their proper folding and trafficking within cells. This property is particularly valuable in therapeutic contexts involving protein misfolding diseases such as cystic fibrosis and certain neurodegenerative disorders .
Case Studies
Several clinical studies have explored the efficacy of N-CP-DNJ in various therapeutic contexts:
- Diabetes Management : A clinical trial involving diabetic patients treated with N-CP-DNJ showed a significant reduction in postprandial blood glucose levels compared to placebo controls.
- Antiviral Efficacy : In animal models infected with DENV, treatment with N-CP-DNJ resulted in reduced viral loads and improved survival rates, indicating its potential as an antiviral agent .
Pharmacokinetics
Pharmacokinetic studies suggest that N-CP-DNJ has favorable absorption characteristics when administered orally. Its bioavailability is enhanced by specific formulations that include adjuvants like carboxymethylcellulose sodium (CMCNa), which have been shown to modify absorption rates positively .
Q & A
Basic Research Questions
Q. What is the primary mechanism of action of N-Cyclohexylpropyl Deoxynojirimycin, Hydrochloride in inhibiting glycosidases?
this compound acts as a competitive inhibitor of α-glucosidases, particularly trimming enzymes I and II, which process N-linked glycans. It mimics the transition state of glucose during substrate hydrolysis, binding to the enzyme's active site and preventing glycan maturation. Methodologically, this can be validated using enzyme kinetics assays (e.g., Michaelis-Menten analysis with varying substrate concentrations) and structural studies like X-ray crystallography to visualize inhibitor-enzyme interactions .
Q. What are the common experimental applications of this compound in glycobiology research?
Researchers employ this compound to:
- Study N-linked glycan processing in endoplasmic reticulum-associated degradation (ERAD) pathways.
- Investigate viral glycoprotein maturation (e.g., Dengue, Influenza A) by disrupting host-cell glycosylation.
- Modulate trehalase activity in comparative enzymology studies (e.g., insect vs. mammalian enzymes). Key techniques include viral plaque reduction assays, glycan profiling via mass spectrometry, and kinetic analysis of trehalase inhibition .
Q. How is N-Cyclohexylpropyl Deoxynojirimycin synthesized, and what quality control measures are used?
Industrial synthesis involves regioselective oxidation of aminosorbitol using Gluconobacter oxydans, followed by N-alkylation with cyclohexylpropyl groups. Critical quality control steps include:
- HPLC purity analysis (e.g., C18 columns, UV detection at 210 nm).
- Chiral chromatography to confirm stereochemical integrity.
- NMR spectroscopy for structural validation (e.g., ¹H/¹³C spectra for glycosidic bond confirmation) .
Advanced Research Questions
Q. How can researchers design experiments to evaluate the compound’s antiviral efficacy against RNA viruses?
- In vitro models : Use viral replication assays in Vero or MDCK cells infected with Dengue/Influenza A.
- Endpoint quantification : Measure viral RNA load via RT-qPCR or plaque-forming units (PFUs).
- Mechanistic studies : Perform time-of-addition assays to determine if inhibition occurs during viral entry or post-entry glycan processing.
- In vivo validation : Test survival rates in murine models infected with adapted viral strains .
Q. How should researchers address contradictory inhibition data between insect and mammalian trehalases?
Contradictions arise from differences in enzyme active-site conformations. To resolve this:
- Perform comparative enzyme kinetics (e.g., Ki values under varying pH/temperature).
- Use molecular docking simulations to map inhibitor-enzyme interactions (e.g., AutoDock Vina).
- Conduct site-directed mutagenesis on key residues (e.g., catalytic aspartates) to identify structural determinants of inhibition specificity .
Q. What methodologies optimize the extraction of deoxynojirimycin derivatives from natural sources?
Supercritical CO₂ extraction (SC-CO₂) is optimal for bioactive recovery:
- Parameter optimization : Use a 3² factorial design to test pressure (100–200 bar), temperature (40–60°C), and dynamic extraction time (40–80 min).
- Co-solvent selection : Ethanol (10–20% v/v) enhances polarity-matched solubility.
- Validation : Quantify yield via HPLC with evaporative light scattering detection (ELSD) and compare against SC-CO₂ efficiency benchmarks (e.g., >95% recovery) .
Q. How can structure-activity relationships (SAR) guide the design of more potent derivatives?
- Core modifications : Synthesize N-alkylated analogs (e.g., N-butyl, N-benzyl) to assess hydrophobicity effects.
- Functional group addition : Introduce hydroxyl or amine groups to enhance hydrogen bonding with enzyme targets.
- In silico screening : Use molecular dynamics simulations (e.g., GROMACS) to predict binding free energy (ΔG) and prioritize candidates for synthesis .
Q. What strategies assess off-target effects on mammalian glycosylation pathways?
- Glycan profiling : Treat cells with the compound and analyze N-glycans via MALDI-TOF-MS.
- Lectin microarray screening : Identify changes in glycan epitopes (e.g., mannose vs. complex glycans).
- CRISPR-Cas9 screens : Knock out specific glycosidases to determine compensatory pathways .
Q. Methodological Considerations
- Data Contradictions : Cross-validate findings using orthogonal techniques (e.g., enzymatic assays vs. structural modeling).
- Ethical Compliance : Follow biosafety protocols (BSL-2+) for viral studies and IACUC guidelines for animal models.
Propiedades
IUPAC Name |
(3R,5R)-1-(3-cyclohexylpropyl)-2-(hydroxymethyl)piperidine-3,4,5-triol;hydrochloride | |
---|---|---|
Source | PubChem | |
URL | https://pubchem.ncbi.nlm.nih.gov | |
Description | Data deposited in or computed by PubChem | |
InChI |
InChI=1S/C15H29NO4.ClH/c17-10-12-14(19)15(20)13(18)9-16(12)8-4-7-11-5-2-1-3-6-11;/h11-15,17-20H,1-10H2;1H/t12?,13-,14-,15?;/m1./s1 | |
Source | PubChem | |
URL | https://pubchem.ncbi.nlm.nih.gov | |
Description | Data deposited in or computed by PubChem | |
InChI Key |
YTHUDNPTOBWSMJ-RZNUZLNKSA-N | |
Source | PubChem | |
URL | https://pubchem.ncbi.nlm.nih.gov | |
Description | Data deposited in or computed by PubChem | |
Canonical SMILES |
C1CCC(CC1)CCCN2CC(C(C(C2CO)O)O)O.Cl | |
Source | PubChem | |
URL | https://pubchem.ncbi.nlm.nih.gov | |
Description | Data deposited in or computed by PubChem | |
Isomeric SMILES |
C1CCC(CC1)CCCN2C[C@H](C([C@@H](C2CO)O)O)O.Cl | |
Source | PubChem | |
URL | https://pubchem.ncbi.nlm.nih.gov | |
Description | Data deposited in or computed by PubChem | |
Molecular Formula |
C15H30ClNO4 | |
Source | PubChem | |
URL | https://pubchem.ncbi.nlm.nih.gov | |
Description | Data deposited in or computed by PubChem | |
DSSTOX Substance ID |
DTXSID80747401 | |
Record name | (3R,5R)-1-(3-Cyclohexylpropyl)-2-(hydroxymethyl)piperidine-3,4,5-triol--hydrogen chloride (1/1) | |
Source | EPA DSSTox | |
URL | https://comptox.epa.gov/dashboard/DTXSID80747401 | |
Description | DSSTox provides a high quality public chemistry resource for supporting improved predictive toxicology. | |
Molecular Weight |
323.85 g/mol | |
Source | PubChem | |
URL | https://pubchem.ncbi.nlm.nih.gov | |
Description | Data deposited in or computed by PubChem | |
CAS No. |
1221793-31-2 | |
Record name | (3R,5R)-1-(3-Cyclohexylpropyl)-2-(hydroxymethyl)piperidine-3,4,5-triol--hydrogen chloride (1/1) | |
Source | EPA DSSTox | |
URL | https://comptox.epa.gov/dashboard/DTXSID80747401 | |
Description | DSSTox provides a high quality public chemistry resource for supporting improved predictive toxicology. | |
Descargo de responsabilidad e información sobre productos de investigación in vitro
Tenga en cuenta que todos los artículos e información de productos presentados en BenchChem están destinados únicamente con fines informativos. Los productos disponibles para la compra en BenchChem están diseñados específicamente para estudios in vitro, que se realizan fuera de organismos vivos. Los estudios in vitro, derivados del término latino "in vidrio", involucran experimentos realizados en entornos de laboratorio controlados utilizando células o tejidos. Es importante tener en cuenta que estos productos no se clasifican como medicamentos y no han recibido la aprobación de la FDA para la prevención, tratamiento o cura de ninguna condición médica, dolencia o enfermedad. Debemos enfatizar que cualquier forma de introducción corporal de estos productos en humanos o animales está estrictamente prohibida por ley. Es esencial adherirse a estas pautas para garantizar el cumplimiento de los estándares legales y éticos en la investigación y experimentación.