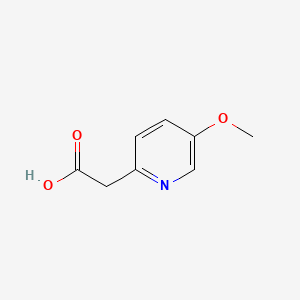
2-(5-Methoxypyridin-2-yl)acetic acid
Descripción general
Descripción
2-(5-Methoxypyridin-2-yl)acetic acid is an organic compound with the molecular formula C8H9NO3. It is a derivative of pyridine, featuring a methoxy group at the 5-position and an acetic acid moiety at the 2-position of the pyridine ring.
Métodos De Preparación
Synthetic Routes and Reaction Conditions: The synthesis of 2-(5-Methoxypyridin-2-yl)acetic acid typically involves the reaction of 2-methoxy-5-nitropyridine with ammonia to form 2-amino-5-methoxypyridine. This intermediate is then subjected to further reactions to introduce the acetic acid moiety . The reaction conditions often include the use of solvents like dichloromethane and catalysts to facilitate the process.
Industrial Production Methods: Industrial production methods for this compound may involve large-scale synthesis using similar reaction pathways as in laboratory settings. The process is optimized for yield and purity, often employing continuous flow reactors and advanced purification techniques to ensure the quality of the final product .
Análisis De Reacciones Químicas
Types of Reactions: 2-(5-Methoxypyridin-2-yl)acetic acid can undergo various chemical reactions, including:
Oxidation: The methoxy group can be oxidized to form corresponding aldehydes or acids.
Reduction: The nitro group in intermediates can be reduced to amines.
Substitution: The hydrogen atoms on the pyridine ring can be substituted with other functional groups under appropriate conditions.
Common Reagents and Conditions: Common reagents used in these reactions include oxidizing agents like potassium permanganate, reducing agents like hydrogen gas or palladium on carbon, and various nucleophiles for substitution reactions. The conditions often involve controlled temperatures and pressures to achieve the desired transformations .
Major Products: The major products formed from these reactions depend on the specific reagents and conditions used. For example, oxidation of the methoxy group can yield methoxy-substituted aldehydes or acids, while reduction of nitro intermediates results in amines .
Aplicaciones Científicas De Investigación
2-(5-Methoxypyridin-2-yl)acetic acid has several scientific research applications:
Chemistry: It serves as an intermediate in the synthesis of various organic compounds, including pharmaceuticals and agrochemicals.
Biology: The compound is used in studies related to enzyme inhibition and receptor binding due to its structural similarity to biologically active molecules.
Industry: Utilized in the production of specialty chemicals and as a building block for more complex molecules
Mecanismo De Acción
The mechanism of action of 2-(5-Methoxypyridin-2-yl)acetic acid involves its interaction with specific molecular targets, such as enzymes or receptors. The methoxy and acetic acid groups play crucial roles in binding to these targets, influencing the compound’s biological activity. The pathways involved may include inhibition of enzyme activity or modulation of receptor signaling, depending on the specific application .
Comparación Con Compuestos Similares
- 2-(5-Methoxypyridin-3-yl)acetic acid
- 2-(4-Methoxypyridin-2-yl)acetic acid
- 2-(5-Methoxypyridin-4-yl)acetic acid
Comparison: Compared to these similar compounds, 2-(5-Methoxypyridin-2-yl)acetic acid is unique due to the specific positioning of the methoxy and acetic acid groups, which can significantly influence its chemical reactivity and biological activity. This uniqueness makes it a valuable compound for targeted research and industrial applications .
Actividad Biológica
2-(5-Methoxypyridin-2-yl)acetic acid (MPAA) is a pyridine derivative that has garnered attention due to its diverse biological activities. This compound features a methoxy group and an acetic acid moiety, which are critical for its interaction with various biological targets. Understanding the biological activity of MPAA involves exploring its mechanisms of action, efficacy in different biological systems, and potential therapeutic applications.
The biological activity of MPAA is primarily attributed to its ability to interact with specific molecular targets, including enzymes and receptors. The methoxy group enhances binding affinity, while the acetic acid moiety may facilitate interactions that modulate enzyme activity or receptor signaling pathways.
Research indicates that MPAA can inhibit certain enzymes, potentially influencing metabolic pathways related to inflammation and cancer progression. Its unique structure allows it to engage in various biochemical interactions, making it a candidate for further pharmacological exploration .
Anticancer Properties
Studies have shown that MPAA exhibits cytotoxic effects against various cancer cell lines. For instance, in vitro assays demonstrated that MPAA can inhibit the proliferation of HepG2 (liver cancer) and MDA-MB-231 (breast cancer) cell lines. The IC50 values for these cell lines were found to be 74.2 μM and 27.1 μM, respectively, indicating moderate cytotoxicity .
Anti-inflammatory Effects
MPAA has also been investigated for its anti-inflammatory properties. The compound's ability to modulate inflammatory pathways may provide therapeutic benefits in conditions characterized by chronic inflammation. Further studies are required to elucidate the specific mechanisms through which MPAA exerts these effects.
Comparative Analysis with Similar Compounds
To understand the uniqueness of MPAA, it is useful to compare it with other pyridine derivatives:
Compound Name | Structure | Biological Activity |
---|---|---|
2-(5-Methoxypyridin-3-yl)acetic acid | Structure | Moderate enzyme inhibition |
2-(4-Methoxypyridin-2-yl)acetic acid | Structure | Varies based on substitution pattern |
2-(5-Methoxypyridin-4-yl)acetic acid | Structure | Unique reactivity and activity profile |
MPAA stands out due to its specific substitution pattern, which influences its chemical reactivity and biological activity compared to other derivatives.
Case Study 1: Cytotoxicity Assessment
In a study assessing the cytotoxic effects of MPAA, researchers evaluated its impact on several cancer cell lines. The results indicated that MPAA effectively inhibited cell growth in a dose-dependent manner, particularly in MDA-MB-231 cells. This suggests potential for MPAA as a lead compound in developing anticancer therapies.
Case Study 2: Enzyme Interaction Studies
Another study focused on the interaction of MPAA with specific enzymes involved in metabolic pathways. The findings revealed that MPAA could inhibit enzyme activity significantly, suggesting a potential role in regulating metabolic disorders or inflammatory responses.
Propiedades
IUPAC Name |
2-(5-methoxypyridin-2-yl)acetic acid | |
---|---|---|
Source | PubChem | |
URL | https://pubchem.ncbi.nlm.nih.gov | |
Description | Data deposited in or computed by PubChem | |
InChI |
InChI=1S/C8H9NO3/c1-12-7-3-2-6(9-5-7)4-8(10)11/h2-3,5H,4H2,1H3,(H,10,11) | |
Source | PubChem | |
URL | https://pubchem.ncbi.nlm.nih.gov | |
Description | Data deposited in or computed by PubChem | |
InChI Key |
LGEKFLLRSSKDBY-UHFFFAOYSA-N | |
Source | PubChem | |
URL | https://pubchem.ncbi.nlm.nih.gov | |
Description | Data deposited in or computed by PubChem | |
Canonical SMILES |
COC1=CN=C(C=C1)CC(=O)O | |
Source | PubChem | |
URL | https://pubchem.ncbi.nlm.nih.gov | |
Description | Data deposited in or computed by PubChem | |
Molecular Formula |
C8H9NO3 | |
Source | PubChem | |
URL | https://pubchem.ncbi.nlm.nih.gov | |
Description | Data deposited in or computed by PubChem | |
Molecular Weight |
167.16 g/mol | |
Source | PubChem | |
URL | https://pubchem.ncbi.nlm.nih.gov | |
Description | Data deposited in or computed by PubChem | |
Synthesis routes and methods
Procedure details
Descargo de responsabilidad e información sobre productos de investigación in vitro
Tenga en cuenta que todos los artículos e información de productos presentados en BenchChem están destinados únicamente con fines informativos. Los productos disponibles para la compra en BenchChem están diseñados específicamente para estudios in vitro, que se realizan fuera de organismos vivos. Los estudios in vitro, derivados del término latino "in vidrio", involucran experimentos realizados en entornos de laboratorio controlados utilizando células o tejidos. Es importante tener en cuenta que estos productos no se clasifican como medicamentos y no han recibido la aprobación de la FDA para la prevención, tratamiento o cura de ninguna condición médica, dolencia o enfermedad. Debemos enfatizar que cualquier forma de introducción corporal de estos productos en humanos o animales está estrictamente prohibida por ley. Es esencial adherirse a estas pautas para garantizar el cumplimiento de los estándares legales y éticos en la investigación y experimentación.