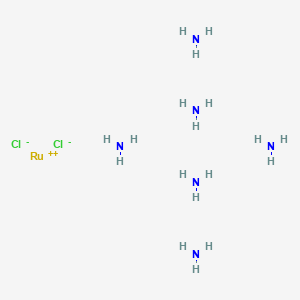
Azane;ruthenium(2+);dichloride
- Haga clic en CONSULTA RÁPIDA para recibir una cotización de nuestro equipo de expertos.
- Con productos de calidad a un precio COMPETITIVO, puede centrarse más en su investigación.
Descripción general
Descripción
Azane;ruthenium(2+);dichloride, also known as hexaammineruthenium(II) chloride, is an inorganic compound with the formula [Ru(NH3)6]Cl2. It is a coordination complex of ruthenium, where the central ruthenium ion is in the +2 oxidation state and is surrounded by six ammonia (azane) ligands. This compound is known for its stability and unique chemical properties, making it a subject of interest in various fields of scientific research .
Métodos De Preparación
Synthetic Routes and Reaction Conditions
Hexaammineruthenium(II) chloride can be synthesized through several methods. One common method involves the reduction of ruthenium trichloride (RuCl3) with hydrogen in the presence of ammonia. The reaction typically occurs in an ethanol solution with platinum black as a catalyst: [ 2RuCl3 + 6NH3 + H2 \rightarrow 2[Ru(NH3)6]Cl2 + 2HCl ]
Another method involves the direct reaction of ruthenium metal with chlorine gas at elevated temperatures: [ Ru + Cl2 \rightarrow RuCl2 ]
Industrial Production Methods
Industrial production of hexaammineruthenium(II) chloride often involves large-scale synthesis using similar methods as described above. The process is optimized for yield and purity, with careful control of reaction conditions such as temperature, pressure, and the concentration of reactants .
Análisis De Reacciones Químicas
Types of Reactions
Hexaammineruthenium(II) chloride undergoes various chemical reactions, including:
Oxidation: The compound can be oxidized to higher oxidation states of ruthenium.
Reduction: It can be reduced to elemental ruthenium.
Substitution: The ammonia ligands can be substituted with other ligands such as phosphines or carbonyls.
Common Reagents and Conditions
Oxidation: Common oxidizing agents include oxygen, chlorine, and nitric acid.
Reduction: Hydrogen gas in the presence of a catalyst like platinum black is commonly used.
Substitution: Ligand exchange reactions often occur in the presence of other ligands and solvents like ethanol or acetonitrile.
Major Products Formed
Oxidation: Higher oxidation state complexes such as [Ru(NH3)6]Cl3.
Reduction: Elemental ruthenium.
Substitution: Various substituted complexes like [Ru(NH3)5Cl]Cl2.
Aplicaciones Científicas De Investigación
Hexaammineruthenium(II) chloride has a wide range of applications in scientific research:
Chemistry: Used as a precursor for the synthesis of other ruthenium complexes and as a catalyst in various chemical reactions.
Biology: Investigated for its potential as an anticancer agent due to its ability to interact with DNA and proteins.
Medicine: Explored for its therapeutic properties, including its use in photodynamic therapy.
Industry: Utilized in the production of advanced materials and as a catalyst in industrial processes.
Mecanismo De Acción
The mechanism of action of hexaammineruthenium(II) chloride involves its interaction with biological molecules such as DNA and proteins. The compound can bind to DNA, causing structural changes that inhibit replication and transcription. It also interacts with proteins, affecting their function and leading to cell death. The exact molecular targets and pathways involved depend on the specific biological context and the nature of the interacting molecules .
Comparación Con Compuestos Similares
Hexaammineruthenium(II) chloride can be compared with other similar compounds such as:
Hexaammineruthenium(III) chloride: Similar structure but with ruthenium in the +3 oxidation state.
Ruthenium(II) chloride: A simpler compound with ruthenium in the +2 oxidation state but without ammonia ligands.
Ruthenium(III) chloride: A common precursor for the synthesis of various ruthenium complexes.
Uniqueness
Hexaammineruthenium(II) chloride is unique due to its stability and the presence of six ammonia ligands, which provide a high degree of coordination and influence its reactivity and interaction with other molecules .
Propiedades
Número CAS |
15305-72-3 |
---|---|
Fórmula molecular |
ClH12N6Ru+ |
Peso molecular |
232.7 g/mol |
Nombre IUPAC |
azanide;ruthenium(8+);chloride |
InChI |
InChI=1S/ClH.6H2N.Ru/h1H;6*1H2;/q;6*-1;+8/p-1 |
Clave InChI |
MHYZHXDLILNYTQ-UHFFFAOYSA-M |
SMILES |
N.N.N.N.N.N.[Cl-].[Cl-].[Ru+2] |
SMILES canónico |
[NH2-].[NH2-].[NH2-].[NH2-].[NH2-].[NH2-].[Cl-].[Ru+8] |
Origen del producto |
United States |
Q1: How does Hexaammineruthenium(II) chloride interact with biological molecules, and what are the implications of these interactions?
A1: Research indicates that Hexaammineruthenium(II) chloride interacts with biological molecules primarily through electron transfer processes. [, , ] For instance, studies using iso-1-cytochrome c variants demonstrated that Hexaammineruthenium(II) chloride acts as an electron donor, influencing the protein's conformational dynamics and modulating electron transfer rates. [, ] This interaction highlights the compound's potential as a probe for investigating electron transfer mechanisms in biological systems.
Q2: Can you elaborate on the role of Hexaammineruthenium(II) chloride in studying conformational changes in proteins?
A2: Hexaammineruthenium(II) chloride proves valuable in studying protein conformational changes due to its electron transfer properties. [, ] Researchers utilized this compound to investigate the alkaline conformational transition in iso-1-cytochrome c variants. [, ] By monitoring the electron transfer kinetics between Hexaammineruthenium(II) chloride and the cytochrome c variants at different pH values, researchers could discern the influence of specific amino acid mutations on the protein's conformational dynamics and the accessibility of different conformational states. This approach enables a deeper understanding of protein structure-function relationships and the factors governing conformational transitions.
Q3: What insights do the provided research papers offer regarding the stability of Hexaammineruthenium(II) chloride under different conditions?
A3: The research highlights that the stability of Hexaammineruthenium(II) chloride can be influenced by factors such as pH and the presence of other molecules. [, ] For example, in a study investigating interactions within sol-gel silica matrixes, Hexaammineruthenium(II) chloride exhibited varying degrees of decomposition depending on the co-encapsulation method and the presence of DNA purines. [] The study observed that adenine could inhibit the oxidation of Hexaammineruthenium(II) chloride, indicating a potential stabilizing effect. These findings emphasize the importance of considering environmental factors when utilizing Hexaammineruthenium(II) chloride in experimental settings.
Q4: How is Hexaammineruthenium(II) chloride employed in microfluidic electrochemical systems, and what advantages does this approach offer?
A4: Research demonstrates the application of Hexaammineruthenium(II) chloride as a redox-active species in microfluidic electrochemical cells. [] Researchers successfully utilized this compound to investigate electrochemical reactions within microfluidic flow cells, showcasing its utility in miniaturized systems. [] The use of microfluidic platforms with integrated Hexaammineruthenium(II) chloride offers advantages such as reduced sample volume, enhanced mass transport, and precise control over experimental parameters, making it a promising approach for various electrochemical studies.
Descargo de responsabilidad e información sobre productos de investigación in vitro
Tenga en cuenta que todos los artículos e información de productos presentados en BenchChem están destinados únicamente con fines informativos. Los productos disponibles para la compra en BenchChem están diseñados específicamente para estudios in vitro, que se realizan fuera de organismos vivos. Los estudios in vitro, derivados del término latino "in vidrio", involucran experimentos realizados en entornos de laboratorio controlados utilizando células o tejidos. Es importante tener en cuenta que estos productos no se clasifican como medicamentos y no han recibido la aprobación de la FDA para la prevención, tratamiento o cura de ninguna condición médica, dolencia o enfermedad. Debemos enfatizar que cualquier forma de introducción corporal de estos productos en humanos o animales está estrictamente prohibida por ley. Es esencial adherirse a estas pautas para garantizar el cumplimiento de los estándares legales y éticos en la investigación y experimentación.