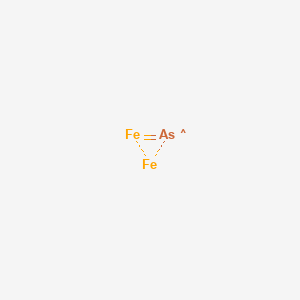
Arseniuro de hierro
- Haga clic en CONSULTA RÁPIDA para recibir una cotización de nuestro equipo de expertos.
- Con productos de calidad a un precio COMPETITIVO, puede centrarse más en su investigación.
Descripción general
Descripción
Iron arsenide is a compound consisting of iron and arsenic. It is known for its unique properties and applications, particularly in the field of superconductivity. Iron arsenide compounds, such as FeAs, FeAs₂, and Fe₂As, have garnered significant interest due to their potential use in various scientific and industrial applications .
Aplicaciones Científicas De Investigación
Iron arsenide has a wide range of scientific research applications, including:
Superconductivity: Iron arsenide compounds are known for their superconducting properties, making them valuable in the development of high-temperature superconductors.
Thermoelectric Materials: Iron arsenide is used in the development of thermoelectric materials, which can convert heat into electricity.
Electronic Devices: The unique electronic properties of iron arsenide make it suitable for use in various electronic devices, including transistors and sensors.
Mecanismo De Acción
Target of Action
Iron arsenide, also known as MFCD00151533, is primarily used in the field of semiconductors , superconductors , and photo optic applications . It is also a key component in the extraction of gold from double refractory gold-arsenic-bearing carbonaceous ores .
Mode of Action
Iron arsenide interacts with its targets through a series of complex reactions. For instance, in the context of gold extraction, iron arsenide plays a crucial role in locking arsenic into iron arsenide phases under reducing atmospheres in the presence of an iron-rich slag . This process is essential for the effective removal of arsenic from arsenic-bearing gold concentrates .
Biochemical Pathways
Arsenic, a component of iron arsenide, is known to undergo various biotransformation processes such as uptake, accumulation, reduction, oxidation, methylation, and demethylation . These processes involve a certain set of genes and proteins
Pharmacokinetics
It is known that iron arsenide is insoluble in water , which could impact its bioavailability and distribution in the body
Result of Action
Arsenic, a component of iron arsenide, is known to have toxic effects on cells
Action Environment
The action of iron arsenide can be influenced by various environmental factors. For instance, the presence of oxalate on the adsorbent surface plays an important role in the recycling of Fe (II)/Fe (III) to minimize the arsenic concentration . Additionally, microbial-mediated redox of iron has an important influence on the environmental behavior of arsenic .
Análisis Bioquímico
Biochemical Properties
Iron arsenide plays a significant role in biochemical reactions . It interacts with various enzymes, proteins, and other biomolecules. The nature of these interactions is complex and multifaceted, involving various biochemical pathways .
Cellular Effects
Iron arsenide has profound effects on various types of cells and cellular processes . It influences cell function, including impacts on cell signaling pathways, gene expression, and cellular metabolism .
Molecular Mechanism
The mechanism of action of iron arsenide is intricate. It exerts its effects at the molecular level, including binding interactions with biomolecules, enzyme inhibition or activation, and changes in gene expression .
Temporal Effects in Laboratory Settings
In laboratory settings, the effects of iron arsenide change over time. Information on the product’s stability, degradation, and any long-term effects on cellular function observed in in vitro or in vivo studies is continually being updated .
Dosage Effects in Animal Models
The effects of iron arsenide vary with different dosages in animal models . Studies have observed threshold effects, as well as toxic or adverse effects at high doses .
Metabolic Pathways
Iron arsenide is involved in various metabolic pathways. It interacts with several enzymes or cofactors, and can affect metabolic flux or metabolite levels .
Transport and Distribution
Iron arsenide is transported and distributed within cells and tissues in a specific manner . It interacts with certain transporters or binding proteins, and can affect its localization or accumulation .
Subcellular Localization
The subcellular localization of iron arsenide and its effects on its activity or function are areas of active research . It could include any targeting signals or post-translational modifications that direct it to specific compartments or organelles .
Métodos De Preparación
Synthetic Routes and Reaction Conditions: Iron arsenide can be synthesized through various methods, including solid-state reactions and chemical vapor deposition. One common method involves the reaction of iron powder with arsenic powder at high temperatures in a sealed tube to prevent oxidation. The reaction typically occurs at temperatures ranging from 600°C to 900°C .
Industrial Production Methods: In industrial settings, iron arsenide is often produced by heating a mixture of iron and arsenic in a controlled atmosphere. This process ensures the formation of the desired iron arsenide phase while minimizing the formation of unwanted by-products. The use of high-purity starting materials and precise control of reaction conditions are crucial for obtaining high-quality iron arsenide .
Análisis De Reacciones Químicas
Types of Reactions: Iron arsenide undergoes various chemical reactions, including oxidation, reduction, and substitution reactions.
Common Reagents and Conditions:
Oxidation: Iron arsenide can be oxidized in the presence of oxygen or other oxidizing agents, leading to the formation of iron oxides and arsenic oxides.
Reduction: Reduction of iron arsenide can be achieved using reducing agents such as hydrogen gas or carbon monoxide, resulting in the formation of elemental iron and arsenic.
Major Products Formed:
Oxidation: Iron oxides (Fe₂O₃, Fe₃O₄) and arsenic oxides (As₂O₃).
Reduction: Elemental iron (Fe) and arsenic (As).
Substitution: Compounds such as iron phosphide (FeP) or iron sulfide (FeS).
Comparación Con Compuestos Similares
Iron arsenide can be compared with other similar compounds, such as:
Gallium Arsenide (GaAs): Gallium arsenide is another compound known for its electronic properties and is widely used in semiconductor devices.
Indium Arsenide (InAs): Indium arsenide is used in high-speed electronic devices and infrared detectors.
Aluminum Arsenide (AlAs): Aluminum arsenide is used in optoelectronic devices and has similar electronic properties to gallium arsenide.
Iron arsenide’s uniqueness lies in its superconducting properties, which are not observed in other arsenide compounds. This makes it a valuable material for research and development in the field of superconductivity .
Propiedades
Número CAS |
12005-88-8 |
---|---|
Fórmula molecular |
AsFe2 |
Peso molecular |
186.61 g/mol |
InChI |
InChI=1S/As.2Fe |
Clave InChI |
FDKAYGUKROYPRO-UHFFFAOYSA-N |
SMILES |
[Fe].[Fe]=[As] |
SMILES canónico |
[Fe].[Fe]=[As] |
Origen del producto |
United States |
Q1: What is the basic structural motif of iron arsenide superconductors?
A1: The key structural element in iron arsenide superconductors is a square lattice of iron atoms, with pnictogen or chalcogen atoms (like arsenic, phosphorus, selenium, or tellurium) situated above and below the iron plane in a tetrahedral coordination. [] This contrasts with the oxygen octahedra found in cuprate superconductors.
Q2: How does the FeAs4 tetrahedron geometry influence superconductivity in iron arsenides?
A2: Research suggests a correlation between the As-Fe-As bond angles in the FeAs4 tetrahedra and the superconducting transition temperature (Tc). More regular, less distorted tetrahedra generally correspond to higher Tc values. [] For instance, LiFeAs exhibits compressed FeAs4 tetrahedra in the basal plane compared to other iron arsenide superconductors, and applying pressure further distorts these tetrahedra while decreasing Tc. []
Q3: Can you elaborate on the structural transitions observed in iron arsenides?
A4: Many iron arsenides undergo a structural transition from a tetragonal to an orthorhombic phase upon cooling. This is often followed by the emergence of antiferromagnetic ordering at even lower temperatures. [, , ] For instance, LaFeAsO undergoes this tetragonal-orthorhombic transition around 160 K, followed by antiferromagnetic ordering near 145 K. []
Q4: How does doping affect the structure and properties of iron arsenides?
A5: Doping plays a crucial role in inducing superconductivity in iron arsenides. For example, BaFe2As2, a non-superconducting parent compound, becomes superconducting upon hole doping with potassium (Ba1-xKxFe2As2). [] This doping suppresses the SDW anomaly present in the parent compound and gives rise to superconductivity. Similarly, electron doping in BaFe2As2, achieved through cobalt or nickel substitution at the iron sites, also induces superconductivity. []
Q5: What is the role of magnetism in iron arsenide superconductors?
A6: The interplay between magnetism and superconductivity is a defining characteristic of iron arsenides. Most undoped parent compounds exhibit antiferromagnetic ordering at low temperatures. [, ] Superconductivity emerges when this magnetic order is suppressed, typically through doping or the application of pressure. [, , ]
Q6: How does the electronic structure of iron arsenides differ from cuprate superconductors?
A7: Unlike cuprate superconductors, which are Mott insulators in their undoped state, iron arsenides are poor metals. [] Additionally, iron arsenides have multiple bands crossing the Fermi level, leading to a more complex electronic structure compared to cuprates, which primarily involve a single band. []
Q7: What is the significance of the "resonance" observed in inelastic neutron scattering experiments on iron arsenides?
A8: The "resonance," a peak in the spin excitation spectrum observed in materials like BaFe1.9Ni0.1As2, provides strong evidence for an unconventional pairing mechanism in iron arsenide superconductors. [] The resonance energy is closely tied to the superconducting gap energy, suggesting that spin fluctuations play a vital role in mediating electron pairing. []
Q8: What experimental techniques are used to study the electronic structure of iron arsenides?
A9: Various techniques are employed to probe the electronic structure, including angle-resolved photoemission spectroscopy (ARPES), [] inelastic neutron scattering, [, , ] and optical spectroscopy. [, ] These techniques help researchers understand the Fermi surface topology, the superconducting gap structure, and the nature of electronic correlations in these materials.
Q9: What synthesis methods are commonly used to prepare iron arsenide superconductors?
A10: High-temperature solid-state reactions are frequently employed to synthesize iron arsenides. [, , ] This often involves sealing precursor materials in evacuated ampoules and subjecting them to controlled heating profiles. High-pressure synthesis methods, sometimes coupled with rapid quenching processes, are also utilized, particularly for synthesizing compounds with heavier rare-earth elements like HoFeAsO1-δ. []
Q10: Are there any challenges associated with synthesizing these materials?
A11: Achieving the desired stoichiometry and phase purity can be challenging. For instance, in the Sr1-xNaxFe2As2 system, the sodium content achievable through conventional solid-state synthesis is limited (x = 0.4). [] Higher sodium concentrations lead to the formation of impurity phases like elemental iron. []
Q11: What are the potential applications of iron arsenide superconductors?
A11: While still in the research phase, iron arsenide superconductors hold promise for various applications:
Descargo de responsabilidad e información sobre productos de investigación in vitro
Tenga en cuenta que todos los artículos e información de productos presentados en BenchChem están destinados únicamente con fines informativos. Los productos disponibles para la compra en BenchChem están diseñados específicamente para estudios in vitro, que se realizan fuera de organismos vivos. Los estudios in vitro, derivados del término latino "in vidrio", involucran experimentos realizados en entornos de laboratorio controlados utilizando células o tejidos. Es importante tener en cuenta que estos productos no se clasifican como medicamentos y no han recibido la aprobación de la FDA para la prevención, tratamiento o cura de ninguna condición médica, dolencia o enfermedad. Debemos enfatizar que cualquier forma de introducción corporal de estos productos en humanos o animales está estrictamente prohibida por ley. Es esencial adherirse a estas pautas para garantizar el cumplimiento de los estándares legales y éticos en la investigación y experimentación.