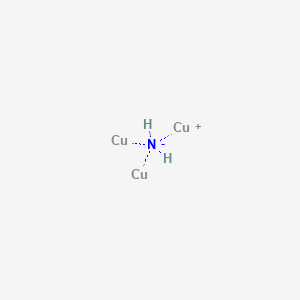
Copper nitride (Cu3N)
Descripción general
Descripción
Copper nitride (Cu3N) is a useful research compound. Its molecular formula is Cu3H2N and its molecular weight is 206.661. The purity is usually 95%.
BenchChem offers high-quality Copper nitride (Cu3N) suitable for many research applications. Different packaging options are available to accommodate customers' requirements. Please inquire for more information about Copper nitride (Cu3N) including the price, delivery time, and more detailed information at info@benchchem.com.
Actividad Biológica
Copper nitride (Cu3N) is a compound that has garnered attention in various fields, particularly due to its unique properties and potential applications. This article explores the biological activity of Cu3N, focusing on its synthesis, structural characteristics, photocatalytic properties, and potential biomedical applications.
Copper nitride is a metastable semiconductor with a crystal structure that exhibits interesting optical and electronic properties. It can be synthesized through various methods, including reactive sputtering and chemical vapor deposition. The biological activity of Cu3N is primarily linked to its photocatalytic capabilities and its interactions at the cellular level.
2. Synthesis of Copper Nitride
Cu3N can be synthesized using several methods, which influence its morphology and properties:
- Reactive Sputtering : This method produces thin films of Cu3N with varying structural orientations. The films exhibit conductivity and optical absorption properties suitable for photocatalytic applications .
- Chemical Methods : Techniques such as the reaction of copper(II) acetate with ammonia in long-chain alcohols yield nanoparticles with different morphologies, including nanoflowers and nanocubes .
3. Structural Properties
The structural characteristics of Cu3N are crucial for its biological activity:
- Crystal Structure : Cu3N typically adopts a ReO3-type structure with a lattice constant ranging from 3.808 to 3.828 Å . The presence of vacancies and self-doping in the crystal lattice enhances its photocatalytic efficiency.
- Optical Properties : Cu3N has an optical band gap of approximately 2.0 eV, which allows it to absorb visible light effectively .
4. Photocatalytic Activity
One of the most significant aspects of Cu3N's biological activity is its photocatalytic properties:
- Degradation of Pollutants : Cu3N has shown remarkable efficiency in degrading organic pollutants such as methyl orange, achieving degradation ratios exceeding 99% within 30 minutes under UV light exposure . This capability is attributed to the generation of hydroxyl radicals (), which are highly reactive oxidizing agents.
Pollutant | Degradation Ratio | Time (min) |
---|---|---|
Methyl Orange | 99.5% | 30 |
5. Biological Applications
The biological activity of Cu3N extends beyond environmental applications:
- Antimicrobial Properties : Preliminary studies suggest that Cu3N exhibits antimicrobial effects, making it a candidate for biomedical applications such as coatings for medical devices or wound dressings.
- Cellular Interactions : Research into the cellular interactions of Cu3N nanoparticles indicates potential cytotoxic effects on certain cancer cell lines while promoting cell viability in others, suggesting a dual role in therapeutic applications .
6. Case Studies and Research Findings
Several studies have highlighted the potential applications and implications of Cu3N in biology:
- A study demonstrated that Cu3N nanoparticles could effectively inhibit bacterial growth, indicating their potential use in antimicrobial coatings .
- Another investigation focused on the photocatalytic degradation of pollutants by Cu3N, emphasizing its ability to mineralize organic contaminants into harmless substances .
7. Conclusion
Copper nitride (Cu3N) presents a promising avenue for research due to its unique properties and biological activity. Its effective photocatalytic capabilities position it as an essential material for environmental remediation, while its potential antimicrobial properties open new possibilities in biomedical applications. Continued research into the synthesis methods and biological interactions will further elucidate the full scope of Cu3N's utility.
Aplicaciones Científicas De Investigación
Applications in Electronics
2.1 Thin-Film Transistors and Integrated Circuits
Cu₃N has been explored as a material for thin-film transistors (TFTs) and integrated circuits due to its semiconducting properties. The ability to control its electrical conductivity through doping allows for the development of both n-type and p-type materials, which are essential for creating complementary metal-oxide-semiconductor (CMOS) circuits .
2.2 Resistive Random Access Memory
Recent studies have indicated that Cu₃N can be utilized in resistive random access memory (ReRAM) devices. Its resistive switching behavior is attributed to the formation and dissolution of conductive filaments within the material, making it a promising candidate for next-generation memory technologies .
Energy Storage Applications
3.1 Lithium-Ion Batteries
Cu₃N has shown potential as an electrode material in lithium-ion batteries, demonstrating excellent performance in terms of capacity and cycle stability. Its high electrochemical activity facilitates efficient charge storage, which is critical for enhancing battery performance .
3.2 Supercapacitors
The compound’s unique properties also make it suitable for supercapacitor applications. Cu₃N can be integrated into supercapacitor designs to improve energy density and power delivery capabilities due to its favorable charge transport characteristics .
Solar Energy Conversion
4.1 Photovoltaic Applications
Copper nitride is emerging as a promising light absorber material for thin-film solar cells. The tunable band gap allows for optimization in solar energy conversion efficiency. Studies have shown that Cu₃N thin films can be fabricated using various deposition techniques such as reactive magnetron sputtering, yielding films with suitable optical properties for photovoltaic applications .
Parameter | Value |
---|---|
Band Gap Energy | 1.4 - 1.8 eV |
Preferred Orientation | (100) to (111) |
Deposition Technique | Reactive RF Magnetron Sputtering |
Substrate Types | Glass, Silicon |
Case Studies
5.1 Fabrication Techniques
A study conducted on the fabrication of Cu₃N thin films via reactive radio-frequency magnetron sputtering highlighted the effects of RF power on film properties. The results indicated that increasing RF power improved the structural quality and optical characteristics of the films, making them more suitable as solar absorbers .
5.2 Performance in Solar Cells
In another case study, Cu₃N was evaluated as a solar absorber material where it displayed strong absorption characteristics in the visible range, confirming its potential as an alternative to traditional silicon-based solar cells .
Propiedades
IUPAC Name |
azanide;copper;copper(1+) | |
---|---|---|
Source | PubChem | |
URL | https://pubchem.ncbi.nlm.nih.gov | |
Description | Data deposited in or computed by PubChem | |
InChI |
InChI=1S/3Cu.H2N/h;;;1H2/q;;+1;-1 | |
Source | PubChem | |
URL | https://pubchem.ncbi.nlm.nih.gov | |
Description | Data deposited in or computed by PubChem | |
InChI Key |
DOIHHHHNLGDDRE-UHFFFAOYSA-N | |
Source | PubChem | |
URL | https://pubchem.ncbi.nlm.nih.gov | |
Description | Data deposited in or computed by PubChem | |
Canonical SMILES |
[NH2-].[Cu].[Cu].[Cu+] | |
Source | PubChem | |
URL | https://pubchem.ncbi.nlm.nih.gov | |
Description | Data deposited in or computed by PubChem | |
Molecular Formula |
Cu3H2N | |
Source | PubChem | |
URL | https://pubchem.ncbi.nlm.nih.gov | |
Description | Data deposited in or computed by PubChem | |
Molecular Weight |
206.66 g/mol | |
Source | PubChem | |
URL | https://pubchem.ncbi.nlm.nih.gov | |
Description | Data deposited in or computed by PubChem | |
CAS No. |
1308-80-1 | |
Record name | Copper nitride (Cu3N) | |
Source | ChemIDplus | |
URL | https://pubchem.ncbi.nlm.nih.gov/substance/?source=chemidplus&sourceid=0001308801 | |
Description | ChemIDplus is a free, web search system that provides access to the structure and nomenclature authority files used for the identification of chemical substances cited in National Library of Medicine (NLM) databases, including the TOXNET system. | |
Record name | Copper nitride (Cu3N) | |
Source | EPA Chemicals under the TSCA | |
URL | https://www.epa.gov/chemicals-under-tsca | |
Description | EPA Chemicals under the Toxic Substances Control Act (TSCA) collection contains information on chemicals and their regulations under TSCA, including non-confidential content from the TSCA Chemical Substance Inventory and Chemical Data Reporting. | |
Record name | Tricopper nitride | |
Source | European Chemicals Agency (ECHA) | |
URL | https://echa.europa.eu/substance-information/-/substanceinfo/100.013.784 | |
Description | The European Chemicals Agency (ECHA) is an agency of the European Union which is the driving force among regulatory authorities in implementing the EU's groundbreaking chemicals legislation for the benefit of human health and the environment as well as for innovation and competitiveness. | |
Explanation | Use of the information, documents and data from the ECHA website is subject to the terms and conditions of this Legal Notice, and subject to other binding limitations provided for under applicable law, the information, documents and data made available on the ECHA website may be reproduced, distributed and/or used, totally or in part, for non-commercial purposes provided that ECHA is acknowledged as the source: "Source: European Chemicals Agency, http://echa.europa.eu/". Such acknowledgement must be included in each copy of the material. ECHA permits and encourages organisations and individuals to create links to the ECHA website under the following cumulative conditions: Links can only be made to webpages that provide a link to the Legal Notice page. | |
Descargo de responsabilidad e información sobre productos de investigación in vitro
Tenga en cuenta que todos los artículos e información de productos presentados en BenchChem están destinados únicamente con fines informativos. Los productos disponibles para la compra en BenchChem están diseñados específicamente para estudios in vitro, que se realizan fuera de organismos vivos. Los estudios in vitro, derivados del término latino "in vidrio", involucran experimentos realizados en entornos de laboratorio controlados utilizando células o tejidos. Es importante tener en cuenta que estos productos no se clasifican como medicamentos y no han recibido la aprobación de la FDA para la prevención, tratamiento o cura de ninguna condición médica, dolencia o enfermedad. Debemos enfatizar que cualquier forma de introducción corporal de estos productos en humanos o animales está estrictamente prohibida por ley. Es esencial adherirse a estas pautas para garantizar el cumplimiento de los estándares legales y éticos en la investigación y experimentación.