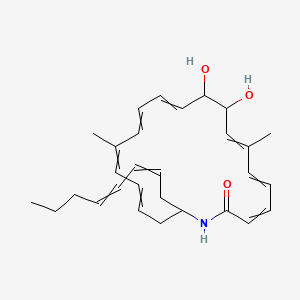
Heronamide C
- Haga clic en CONSULTA RÁPIDA para recibir una cotización de nuestro equipo de expertos.
- Con productos de calidad a un precio COMPETITIVO, puede centrarse más en su investigación.
Descripción general
Descripción
Heronamide C is an unusual polyene macrocyclic lactam containing two isolated tetraene and triene chromophores . It was found to induce a unique reversible vacuolisation of mammalian tumor cells by an as yet unidentified mechanism . It is a new polyketide macrolactam from an Australian marine-derived Streptomyces sp .
Synthesis Analysis
A highly modular synthetic strategy to the Heronamide C-type polyene macrolactams was established by synthesizing 8-deoxyheronamide C . This strategy enabled not only the total synthesis of 8-deoxyheronamide C but also the unified synthesis of four heronamide-like molecules named “heronamidoids” .Molecular Structure Analysis
Structures were assigned to the heronamides on the basis of detailed spectroscopic analysis, chemical derivatization, and biosynthetic considerations . A conformational and reactivity analysis of the heronamidoids clarified that the C19 stereochemistry mainly affected the conformation of the amide linkage, resulting in the change of alignment of two polyene units and reactivity towards photochemical [6π+6π] cycloaddition .Chemical Reactions Analysis
A plausible biosynthetic pathway is proposed in which key carbocyclic ring transformations proceed via an unprecedented synchronized tandem electrocyclization . The C8,C9-diol moiety is important for the conversion to the heronamide A-type skeleton from the Heronamide C skeleton .Physical And Chemical Properties Analysis
Heronamide C has a molecular formula of C29H39NO3 and a formula weight of 449.6 . It is a solid and is soluble in DMSO but unstable in methanol .Aplicaciones Científicas De Investigación
Cancer Research
Heronamide C has been noted to induce a unique reversible vacuolisation of mammalian tumor cells, suggesting potential applications in cancer research or treatment .
Antifungal Activity
The compound has shown antifungal properties, with studies highlighting the importance of specific structural features for its biological activity .
Mecanismo De Acción
Heronamide C, also known as “9,10-Dihydroxy-7,15-dimethyl-20-octa-2,4-dienyl-1-azacycloicosa-3,5,7,11,13,15,17-heptaen-2-one”, is a polyketide macrolactam antifungal agent synthesized by marine Actinomycetes . This article provides a comprehensive analysis of its mechanism of action.
Target of Action
Heronamide C primarily targets the cell membranes of eukaryotic cells . It exhibits selective toxicity by inhibiting growth and promoting abnormal cell wall material accumulation in wild-type fission yeast cells .
Mode of Action
Heronamide C interacts with its targets by binding to liposomes consisting of lipids having saturated hydrocarbon chains in an irreversible manner . This interaction leads to changes in cell wall morphology .
Biochemical Pathways
It is known that the compound is biosynthesized from various β-amino acid starter units, contributing to its structural diversity . The β-amino group of the β-amino acid is essential for the formation of their characteristic macrocyclic structures .
Pharmacokinetics
Its ability to bind irreversibly to lipids suggests that it may have unique pharmacokinetic properties that contribute to its bioavailability .
Result of Action
Heronamide C’s action results in the inhibition of growth and the promotion of abnormal cell wall material accumulation in wild-type fission yeast cells . It also induces morphological alterations in mammalian cells .
Action Environment
The action, efficacy, and stability of Heronamide C can be influenced by environmental factors. For instance, the compound’s interaction with cell membrane lipids suggests that the lipid composition of the target cells could impact its efficacy . .
Safety and Hazards
Direcciones Futuras
The biosynthesis of Heronamide C provides a framework for the assignment of complete relative configurations across all heronamides, and inspires an attractive biomimetic strategy for future total syntheses . It may have potential antifungal activities due to its saturated hydrocarbon chain and its ability to be inserted into the phospholipid bilayer .
Propiedades
IUPAC Name |
9,10-dihydroxy-7,15-dimethyl-20-octa-2,4-dienyl-1-azacycloicosa-3,5,7,11,13,15,17-heptaen-2-one |
Source
|
---|---|---|
Source | PubChem | |
URL | https://pubchem.ncbi.nlm.nih.gov | |
Description | Data deposited in or computed by PubChem | |
InChI |
InChI=1S/C29H39NO3/c1-4-5-6-7-8-9-19-26-20-13-10-16-24(2)17-11-14-21-27(31)28(32)23-25(3)18-12-15-22-29(33)30-26/h6-18,21-23,26-28,31-32H,4-5,19-20H2,1-3H3,(H,30,33) |
Source
|
Source | PubChem | |
URL | https://pubchem.ncbi.nlm.nih.gov | |
Description | Data deposited in or computed by PubChem | |
InChI Key |
QUZNSWBEDCHESP-UHFFFAOYSA-N |
Source
|
Source | PubChem | |
URL | https://pubchem.ncbi.nlm.nih.gov | |
Description | Data deposited in or computed by PubChem | |
Canonical SMILES |
CCCC=CC=CCC1CC=CC=C(C=CC=CC(C(C=C(C=CC=CC(=O)N1)C)O)O)C |
Source
|
Source | PubChem | |
URL | https://pubchem.ncbi.nlm.nih.gov | |
Description | Data deposited in or computed by PubChem | |
Molecular Formula |
C29H39NO3 |
Source
|
Source | PubChem | |
URL | https://pubchem.ncbi.nlm.nih.gov | |
Description | Data deposited in or computed by PubChem | |
Molecular Weight |
449.6 g/mol |
Source
|
Source | PubChem | |
URL | https://pubchem.ncbi.nlm.nih.gov | |
Description | Data deposited in or computed by PubChem | |
Q & A
Q1: What is the primary biological activity of Heronamide C and how does it interact with its target?
A1: Heronamide C is a potent antifungal agent. [] Research suggests that it exerts its antifungal effects by binding to cell membranes, specifically targeting saturated hydrocarbon chains within lipid membranes. [] This interaction disrupts membrane integrity and microdomain structure, ultimately leading to abnormal cell wall morphology in susceptible organisms like fission yeast. []
Q2: How important is the C16-C17 double bond for Heronamide C's antifungal activity?
A2: The C16-C17 double bond in Heronamide C is crucial for its antifungal activity. Studies using a synthetic analog, 16,17-Dihydroheronamide C (lacking the double bond), showed a significant decrease in antifungal potency. [, ] This highlights the essential role of this structural feature in Heronamide C's mechanism of action.
Q3: What is the significance of Heronamide C's stereochemistry for its biological activity?
A3: Heronamide C exhibits chiral recognition with cell membrane components, indicating that its three-dimensional structure is essential for its biological activity. [, ] Further research with the synthetic enantiomer, ent-Heronamide C, confirmed the importance of stereochemistry, as it also exhibited reduced antifungal activity. [, ] This suggests a specific interaction with a chiral target within the cell membrane.
Q4: How does the structure of Heronamide C's hydrocarbon tail influence its activity?
A4: Comparing Heronamide C with another related polyene macrolactam, BE-14106, revealed that the hydrocarbon tail structure significantly impacts antifungal activity. [] BE-14106, possessing one less acetate unit in its hydrocarbon tail compared to Heronamide C, was found to be four times less potent against fission yeast. [] This suggests that the specific length and composition of the hydrocarbon tail are important for optimal interaction with target membranes.
Q5: What is known about the biosynthesis of Heronamide C?
A5: Heronamide C is produced by marine-derived Streptomyces species. [, ] Genomic analysis of Streptomyces sp. TP-A0871 revealed the presence of a biosynthetic gene cluster (BGC) responsible for Heronamide C production. [] This BGC is suggested to utilize polyketide synthase (PKS) machinery for assembling the molecule's core structure. [, ] Interestingly, Heronamides A and B, two related compounds, are formed through spontaneous thermal [6π + 4π] and photochemical [6π + 6π] intramolecular cycloadditions of Heronamide C. [, ] This highlights an unusual biosynthetic pathway where the final products are generated through non-enzymatic modifications of a precursor molecule.
Q6: What are the structural features of Heronamide C?
A6: Heronamide C is a 20-membered polyene macrolactam. [, ] While its exact molecular formula and weight are not specified in the provided abstracts, its structure consists of two polyene units connected by an amide linkage, with a macrocyclic ring incorporating a C8,C9-diol moiety and a hydrocarbon tail. [] The stereochemistry of specific carbons, particularly C19 and the two hydroxylated methine carbons, is crucial for its activity. [, ]
Descargo de responsabilidad e información sobre productos de investigación in vitro
Tenga en cuenta que todos los artículos e información de productos presentados en BenchChem están destinados únicamente con fines informativos. Los productos disponibles para la compra en BenchChem están diseñados específicamente para estudios in vitro, que se realizan fuera de organismos vivos. Los estudios in vitro, derivados del término latino "in vidrio", involucran experimentos realizados en entornos de laboratorio controlados utilizando células o tejidos. Es importante tener en cuenta que estos productos no se clasifican como medicamentos y no han recibido la aprobación de la FDA para la prevención, tratamiento o cura de ninguna condición médica, dolencia o enfermedad. Debemos enfatizar que cualquier forma de introducción corporal de estos productos en humanos o animales está estrictamente prohibida por ley. Es esencial adherirse a estas pautas para garantizar el cumplimiento de los estándares legales y éticos en la investigación y experimentación.