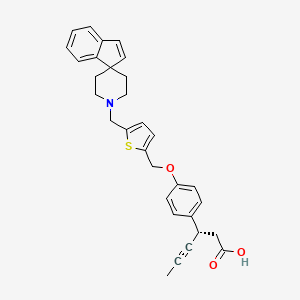
GPR40 Activator 1
Descripción general
Descripción
El Activador GPR40 1 es un compuesto que actúa como agonista del receptor acoplado a proteína G 40 (GPR40), también conocido como receptor 1 de ácidos grasos libres. Este receptor se expresa principalmente en las células beta pancreáticas y juega un papel crucial en la mejora de la secreción de insulina en respuesta a niveles elevados de glucosa. El Activador GPR40 1 ha mostrado potencial en el tratamiento de la diabetes tipo 2 al mejorar la homeostasis de la glucosa y la sensibilidad a la insulina .
Aplicaciones Científicas De Investigación
El Activador GPR40 1 tiene una amplia gama de aplicaciones de investigación científica, incluyendo:
Mecanismo De Acción
El Activador GPR40 1 ejerce sus efectos al unirse y activar GPR40, un receptor que se expresa predominantemente en las células beta pancreáticas. Tras la activación, GPR40 desencadena una cascada de señalización que involucra la activación de la fosfolipasa C, lo que lleva a la hidrólisis de los fosfolípidos de membrana y la generación de inositol-1,4,5-trifosfato y diacilglicerol . Esto da como resultado un aumento en los niveles de calcio intracelular, lo que a su vez mejora la secreción de insulina de una manera dependiente de la glucosa .
Safety and Hazards
Métodos De Preparación
Rutas de Síntesis y Condiciones de Reacción: La síntesis del Activador GPR40 1 implica múltiples pasos, incluyendo la formación de intermediarios clave y sus reacciones de acoplamiento subsiguientes. Una ruta sintética común incluye el uso de grupos alquino y azida, que experimentan una cicloadición azida-alquino catalizada por cobre (CuAAc) para formar el producto final . Las condiciones de reacción típicamente implican el uso de sulfato de cobre y ascorbato de sodio como catalizadores, y la reacción se lleva a cabo en un disolvente adecuado como dimetilsulfóxido o tetrahidrofurano.
Métodos de Producción Industrial: La producción industrial del Activador GPR40 1 sigue rutas sintéticas similares pero a mayor escala. El proceso involucra la optimización de las condiciones de reacción para garantizar un alto rendimiento y pureza. Esto incluye el control preciso de la temperatura, la presión y el tiempo de reacción. El producto final se purifica luego mediante técnicas como la recristalización o la cromatografía para alcanzar el nivel de pureza deseado .
Análisis De Reacciones Químicas
Tipos de Reacciones: El Activador GPR40 1 experimenta varias reacciones químicas, incluyendo:
Oxidación: El compuesto puede oxidarse bajo condiciones específicas para formar derivados oxidados.
Reducción: También puede experimentar reacciones de reducción para producir formas reducidas.
Sustitución: El Activador GPR40 1 puede participar en reacciones de sustitución, donde los grupos funcionales son reemplazados por otros grupos.
Reactivos y Condiciones Comunes:
Oxidación: Los agentes oxidantes comunes incluyen permanganato de potasio y trióxido de cromo.
Reducción: Se utilizan agentes reductores como hidruro de aluminio y litio e hidruro de sodio y boro.
Sustitución: Las condiciones para las reacciones de sustitución varían dependiendo de los grupos funcionales involucrados, pero a menudo incluyen el uso de catalizadores y solventes apropiados.
Productos Principales: Los productos principales formados a partir de estas reacciones dependen de los reactivos y condiciones específicas utilizados. Por ejemplo, la oxidación puede producir derivados oxidados con grupos funcionales adicionales que contienen oxígeno, mientras que la reducción puede producir formas más simples, hidrogenadas del compuesto .
Comparación Con Compuestos Similares
El Activador GPR40 1 es parte de una familia de compuestos conocidos como agonistas del receptor de ácidos grasos libres. Los compuestos similares incluyen:
Activador GPR40 2: Otro agonista con efectos similares en la secreción de insulina.
Activador GPR40 3: Un compuesto con una estructura química ligeramente diferente pero actividad biológica similar.
Activador GPR40 4: Conocido por su mayor potencia y selectividad para GPR40 en comparación con otros activadores.
Singularidad: El Activador GPR40 1 es único en su afinidad de unión específica y perfil de activación para GPR40. Se ha demostrado que mejora eficazmente la secreción de insulina y mejora la homeostasis de la glucosa, lo que lo convierte en un candidato prometedor para el tratamiento de la diabetes tipo 2 .
Propiedades
IUPAC Name |
(3S)-3-[4-[[5-(spiro[indene-1,4'-piperidine]-1'-ylmethyl)thiophen-2-yl]methoxy]phenyl]hex-4-ynoic acid | |
---|---|---|
Source | PubChem | |
URL | https://pubchem.ncbi.nlm.nih.gov | |
Description | Data deposited in or computed by PubChem | |
InChI |
InChI=1S/C31H31NO3S/c1-2-5-25(20-30(33)34)23-8-10-26(11-9-23)35-22-28-13-12-27(36-28)21-32-18-16-31(17-19-32)15-14-24-6-3-4-7-29(24)31/h3-4,6-15,25H,16-22H2,1H3,(H,33,34)/t25-/m0/s1 | |
Source | PubChem | |
URL | https://pubchem.ncbi.nlm.nih.gov | |
Description | Data deposited in or computed by PubChem | |
InChI Key |
YSVQUSMRABAJAR-VWLOTQADSA-N | |
Source | PubChem | |
URL | https://pubchem.ncbi.nlm.nih.gov | |
Description | Data deposited in or computed by PubChem | |
Canonical SMILES |
CC#CC(CC(=O)O)C1=CC=C(C=C1)OCC2=CC=C(S2)CN3CCC4(CC3)C=CC5=CC=CC=C45 | |
Source | PubChem | |
URL | https://pubchem.ncbi.nlm.nih.gov | |
Description | Data deposited in or computed by PubChem | |
Isomeric SMILES |
CC#C[C@@H](CC(=O)O)C1=CC=C(C=C1)OCC2=CC=C(S2)CN3CCC4(CC3)C=CC5=CC=CC=C45 | |
Source | PubChem | |
URL | https://pubchem.ncbi.nlm.nih.gov | |
Description | Data deposited in or computed by PubChem | |
Molecular Formula |
C31H31NO3S | |
Source | PubChem | |
URL | https://pubchem.ncbi.nlm.nih.gov | |
Description | Data deposited in or computed by PubChem | |
Molecular Weight |
497.6 g/mol | |
Source | PubChem | |
URL | https://pubchem.ncbi.nlm.nih.gov | |
Description | Data deposited in or computed by PubChem | |
Retrosynthesis Analysis
AI-Powered Synthesis Planning: Our tool employs the Template_relevance Pistachio, Template_relevance Bkms_metabolic, Template_relevance Pistachio_ringbreaker, Template_relevance Reaxys, Template_relevance Reaxys_biocatalysis model, leveraging a vast database of chemical reactions to predict feasible synthetic routes.
One-Step Synthesis Focus: Specifically designed for one-step synthesis, it provides concise and direct routes for your target compounds, streamlining the synthesis process.
Accurate Predictions: Utilizing the extensive PISTACHIO, BKMS_METABOLIC, PISTACHIO_RINGBREAKER, REAXYS, REAXYS_BIOCATALYSIS database, our tool offers high-accuracy predictions, reflecting the latest in chemical research and data.
Strategy Settings
Precursor scoring | Relevance Heuristic |
---|---|
Min. plausibility | 0.01 |
Model | Template_relevance |
Template Set | Pistachio/Bkms_metabolic/Pistachio_ringbreaker/Reaxys/Reaxys_biocatalysis |
Top-N result to add to graph | 6 |
Feasible Synthetic Routes
Descargo de responsabilidad e información sobre productos de investigación in vitro
Tenga en cuenta que todos los artículos e información de productos presentados en BenchChem están destinados únicamente con fines informativos. Los productos disponibles para la compra en BenchChem están diseñados específicamente para estudios in vitro, que se realizan fuera de organismos vivos. Los estudios in vitro, derivados del término latino "in vidrio", involucran experimentos realizados en entornos de laboratorio controlados utilizando células o tejidos. Es importante tener en cuenta que estos productos no se clasifican como medicamentos y no han recibido la aprobación de la FDA para la prevención, tratamiento o cura de ninguna condición médica, dolencia o enfermedad. Debemos enfatizar que cualquier forma de introducción corporal de estos productos en humanos o animales está estrictamente prohibida por ley. Es esencial adherirse a estas pautas para garantizar el cumplimiento de los estándares legales y éticos en la investigación y experimentación.