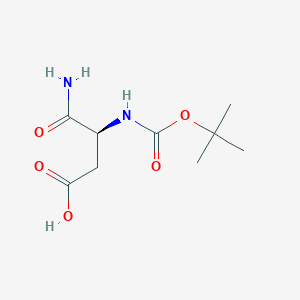
Boc-Asp-NH2
Descripción general
Descripción
Boc-Asp-NH2, also known as Nα-(tert-butoxycarbonyl)-aspartic acid amide, is a chemical compound with the molecular formula C9H16N2O5 and a molecular weight of 232.23 g/mol. It is a derivative of aspartic acid, where the amino group is protected by a tert-butoxycarbonyl (Boc) group. This compound is commonly used in peptide synthesis and other organic synthesis applications due to its stability and reactivity.
Métodos De Preparación
Synthetic Routes and Reaction Conditions
The synthesis of Boc-Asp-NH2 typically involves the protection of the amino group of aspartic acid with a tert-butoxycarbonyl group. This is achieved by reacting aspartic acid with di-tert-butyl dicarbonate (Boc2O) in the presence of a base such as triethylamine or sodium hydroxide . The reaction is usually carried out in an organic solvent like dichloromethane or dimethylformamide at room temperature. The resulting Boc-protected aspartic acid is then converted to its amide form by reacting with ammonia or an amine under suitable conditions .
Industrial Production Methods
In industrial settings, the production of this compound follows similar synthetic routes but on a larger scale. The process involves the use of automated peptide synthesizers and large-scale reactors to ensure efficient and high-yield production. The reaction conditions are optimized to minimize side reactions and maximize the purity of the final product .
Análisis De Reacciones Químicas
Types of Reactions
Boc-Asp-NH2 undergoes various chemical reactions, including:
Deprotection: The Boc group can be removed under acidic conditions using reagents like trifluoroacetic acid (TFA) or hydrochloric acid.
Coupling Reactions: It can participate in peptide bond formation through coupling reactions with other amino acids or peptides using coupling agents like N,N’-dicyclohexylcarbodiimide (DCC) or N-ethyl-N’-(3-dimethylaminopropyl)carbodiimide (EDC).
Substitution Reactions: The amide group can undergo nucleophilic substitution reactions with various electrophiles.
Common Reagents and Conditions
Deprotection: Trifluoroacetic acid, hydrochloric acid.
Coupling: N,N’-dicyclohexylcarbodiimide, N-ethyl-N’-(3-dimethylaminopropyl)carbodiimide.
Substitution: Electrophiles such as alkyl halides or acyl chlorides.
Major Products
Deprotection: Aspartic acid.
Coupling: Peptides or peptide derivatives.
Substitution: Substituted amides or other derivatives.
Aplicaciones Científicas De Investigación
Peptide Synthesis and Modification
Boc-Asp-NH2 serves as a model compound for studying peptide synthesis techniques and modifications. It is often utilized in the development of custom peptides for various research purposes, including drug development and biomolecular studies .
Biological Studies
Research has shown that this compound can influence cellular signaling pathways and protein-protein interactions. Its role in modulating receptor activity makes it a valuable tool for investigating mechanisms of action in biological systems .
Therapeutic Applications
The compound has been explored for potential therapeutic uses, including:
- Drug Delivery Systems : Its ability to form stable complexes with drugs enhances bioavailability and targeting efficiency.
- Peptide-Based Drugs : this compound is being investigated for its efficacy in developing novel peptide-based therapeutics aimed at specific diseases .
Case Study 1: Thermal Synthesis of Polypeptides
A study demonstrated that heating this compound under controlled conditions resulted in the formation of higher molecular weight polypeptides. The research highlighted the stability of intermediates formed during the reaction and provided insights into the thermal behavior of Boc-protected amino acids .
Case Study 2: NMR Studies on Glycopeptides
Nuclear Magnetic Resonance (NMR) studies have been conducted to analyze glycopeptides containing this compound. These studies focused on understanding the conformation and intramolecular interactions within these compounds, revealing significant information about their structural dynamics in solution .
Mecanismo De Acción
The mechanism of action of Boc-Asp-NH2 primarily involves its role as a protected amino acid derivative in peptide synthesis. The Boc group protects the amino group from unwanted reactions during the synthesis process. Upon deprotection, the free amino group can participate in peptide bond formation, allowing for the construction of complex peptide sequences .
Comparación Con Compuestos Similares
Similar Compounds
Boc-Glu-NH2: Boc-protected glutamic acid amide.
Boc-Lys-NH2: Boc-protected lysine amide.
Boc-Ser-NH2: Boc-protected serine amide.
Uniqueness
Boc-Asp-NH2 is unique due to its specific structure and reactivity. The presence of the Boc group provides stability and protection to the amino group, making it suitable for use in peptide synthesis. Additionally, the amide functionality allows for further chemical modifications and applications in various fields .
Actividad Biológica
Boc-Asp-NH2, or N-Boc-L-aspartic acid 1-amide, is a derivative of aspartic acid that has garnered interest in various biological applications due to its unique properties and potential therapeutic benefits. This article explores the biological activity of this compound, focusing on its pharmacological effects, synthesis, and relevant case studies.
Chemical Structure and Properties
This compound has the molecular formula C9H16N2O5 and a molecular weight of 220.24 g/mol. The compound features a tert-butyloxycarbonyl (Boc) protecting group attached to the amino group of aspartic acid, enhancing its stability and solubility in biological systems. The structural modifications aim to improve its pharmacokinetic properties, including absorption and bioavailability.
Pharmacological Activity
1. Hepatoprotective Effects
Research indicates that derivatives of aspartic acid, including this compound, exhibit hepatoprotective properties. Studies have shown that L-aspartic acid can mitigate acute liver injury by modulating inflammatory responses and regulating inflammasome activity. This compound may enhance these effects due to its improved lipophilicity compared to L-aspartic acid, allowing for better cellular uptake and activity in hepatic tissues .
2. Neuroprotective Potential
This compound has also been investigated for its neuroprotective effects. In vitro studies demonstrated that compounds derived from aspartic acid could protect neurons from oxidative stress and excitotoxicity. This suggests potential applications in neurodegenerative diseases where neuronal damage is prevalent .
3. Inhibitory Activity Against Fibrosis
Recent studies have highlighted this compound's role in inhibiting fibrosis-related pathways. Compounds structurally related to this compound showed significant inhibitory rates against collagen production in hepatic cells, indicating a potential therapeutic avenue for treating liver fibrosis .
Case Studies
-
Study on Liver Fibrosis
- Objective : To evaluate the inhibitory effects of this compound on liver fibrosis.
- Method : In vitro assays were conducted using hepatic stellate cells treated with this compound.
- Results : The compound showed a significant reduction in collagen synthesis (up to 69% inhibition) compared to untreated controls.
-
Neuroprotection in Ischemia Models
- Objective : To assess the neuroprotective effects of this compound in models of ischemic injury.
- Method : Primary cortical neurons were exposed to oxygen-glucose deprivation followed by treatment with this compound.
- Results : The compound reduced neuronal death by 50% compared to control groups, suggesting a protective mechanism against ischemic damage.
Data Table: Biological Activities of this compound
Propiedades
IUPAC Name |
(3S)-4-amino-3-[(2-methylpropan-2-yl)oxycarbonylamino]-4-oxobutanoic acid | |
---|---|---|
Source | PubChem | |
URL | https://pubchem.ncbi.nlm.nih.gov | |
Description | Data deposited in or computed by PubChem | |
InChI |
InChI=1S/C9H16N2O5/c1-9(2,3)16-8(15)11-5(7(10)14)4-6(12)13/h5H,4H2,1-3H3,(H2,10,14)(H,11,15)(H,12,13)/t5-/m0/s1 | |
Source | PubChem | |
URL | https://pubchem.ncbi.nlm.nih.gov | |
Description | Data deposited in or computed by PubChem | |
InChI Key |
VKCARTLEXJLJBZ-YFKPBYRVSA-N | |
Source | PubChem | |
URL | https://pubchem.ncbi.nlm.nih.gov | |
Description | Data deposited in or computed by PubChem | |
Canonical SMILES |
CC(C)(C)OC(=O)NC(CC(=O)O)C(=O)N | |
Source | PubChem | |
URL | https://pubchem.ncbi.nlm.nih.gov | |
Description | Data deposited in or computed by PubChem | |
Isomeric SMILES |
CC(C)(C)OC(=O)N[C@@H](CC(=O)O)C(=O)N | |
Source | PubChem | |
URL | https://pubchem.ncbi.nlm.nih.gov | |
Description | Data deposited in or computed by PubChem | |
Molecular Formula |
C9H16N2O5 | |
Source | PubChem | |
URL | https://pubchem.ncbi.nlm.nih.gov | |
Description | Data deposited in or computed by PubChem | |
DSSTOX Substance ID |
DTXSID50427021 | |
Record name | Boc-Asp-NH2 | |
Source | EPA DSSTox | |
URL | https://comptox.epa.gov/dashboard/DTXSID50427021 | |
Description | DSSTox provides a high quality public chemistry resource for supporting improved predictive toxicology. | |
Molecular Weight |
232.23 g/mol | |
Source | PubChem | |
URL | https://pubchem.ncbi.nlm.nih.gov | |
Description | Data deposited in or computed by PubChem | |
CAS No. |
74244-17-0 | |
Record name | Boc-Asp-NH2 | |
Source | EPA DSSTox | |
URL | https://comptox.epa.gov/dashboard/DTXSID50427021 | |
Description | DSSTox provides a high quality public chemistry resource for supporting improved predictive toxicology. | |
Retrosynthesis Analysis
AI-Powered Synthesis Planning: Our tool employs the Template_relevance Pistachio, Template_relevance Bkms_metabolic, Template_relevance Pistachio_ringbreaker, Template_relevance Reaxys, Template_relevance Reaxys_biocatalysis model, leveraging a vast database of chemical reactions to predict feasible synthetic routes.
One-Step Synthesis Focus: Specifically designed for one-step synthesis, it provides concise and direct routes for your target compounds, streamlining the synthesis process.
Accurate Predictions: Utilizing the extensive PISTACHIO, BKMS_METABOLIC, PISTACHIO_RINGBREAKER, REAXYS, REAXYS_BIOCATALYSIS database, our tool offers high-accuracy predictions, reflecting the latest in chemical research and data.
Strategy Settings
Precursor scoring | Relevance Heuristic |
---|---|
Min. plausibility | 0.01 |
Model | Template_relevance |
Template Set | Pistachio/Bkms_metabolic/Pistachio_ringbreaker/Reaxys/Reaxys_biocatalysis |
Top-N result to add to graph | 6 |
Feasible Synthetic Routes
Descargo de responsabilidad e información sobre productos de investigación in vitro
Tenga en cuenta que todos los artículos e información de productos presentados en BenchChem están destinados únicamente con fines informativos. Los productos disponibles para la compra en BenchChem están diseñados específicamente para estudios in vitro, que se realizan fuera de organismos vivos. Los estudios in vitro, derivados del término latino "in vidrio", involucran experimentos realizados en entornos de laboratorio controlados utilizando células o tejidos. Es importante tener en cuenta que estos productos no se clasifican como medicamentos y no han recibido la aprobación de la FDA para la prevención, tratamiento o cura de ninguna condición médica, dolencia o enfermedad. Debemos enfatizar que cualquier forma de introducción corporal de estos productos en humanos o animales está estrictamente prohibida por ley. Es esencial adherirse a estas pautas para garantizar el cumplimiento de los estándares legales y éticos en la investigación y experimentación.