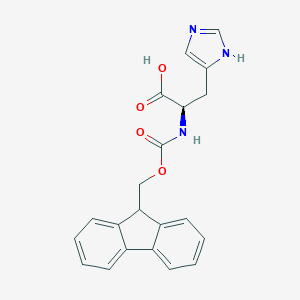
FMOC-D-HIS-OH
Descripción general
Descripción
Nalpha-Fmoc-D-histidine is a biochemical used for proteomics research . It has a molecular formula of C21H19N3O4 and a molecular weight of 377.4 .
Synthesis Analysis
The synthesis of histidine-containing peptides can be achieved through aqueous microwave-assisted solid-phase peptide synthesis using the Fmoc strategy . This method uses water-dispersible 9-fluorenylmethoxycarbonyl-amino acid nanoparticles and is an organic solvent-free, environmentally friendly method for chemical peptide synthesis .Molecular Structure Analysis
The molecular structure of Nalpha-Fmoc-D-histidine is represented by the formula C21H19N3O4 .Chemical Reactions Analysis
Histidine can modulate the assembly behavior of Fmoc diphenylalanine peptide (Fmoc–F–F) and induce enzyme-like catalysis . The presence of histidine rearranges the structure of Fmoc–F–F to assemble nano filaments, resulting in the formation of an active site to mimic peroxidase-like activity that catalyzes ROS generation .Physical And Chemical Properties Analysis
Nalpha-Fmoc-D-histidine appears as an off-white solid . It has a molecular weight of 377.4 . It should be stored at temperatures between -5 °C and 5 °C .Aplicaciones Científicas De Investigación
Síntesis de Péptidos en Fase Sólida (SPPS)
Fmoc-D-His-OH se usa comúnmente como bloque de construcción en SPPS. Permite la incorporación de residuos de histidina en péptidos mientras minimiza la racemización durante las reacciones de acoplamiento y esterificación .
Formación de Hidrogel
Los aminoácidos derivados de Fmoc, incluido this compound, se utilizan para construir hidrogeles autoportantes. Estos hidrogeles tienen posibles aplicaciones biomédicas debido a su biocompatibilidad y propiedades estructurales .
Minimización de la Racemización
El compuesto se sintetiza y utiliza en procesos donde la minimización de la racemización de los residuos de histidina es crucial, particularmente en la producción de péptidos de prueba .
Aplicaciones Biomédicas
Los hidrogeles autoportantes formados a partir de this compound se pueden utilizar en diversas aplicaciones biomédicas, como la ingeniería de tejidos y los sistemas de administración de fármacos .
Estudios Estructurales
Los hidrogeles basados en this compound se pueden estudiar por su ultraestructura y dimensiones, que son similares a las fibrillas amiloides, proporcionando información sobre las enfermedades por mal plegamiento de proteínas .
Ciencia de Materiales
La capacidad del compuesto para formar estructuras estables lo hace valioso en la ciencia de materiales para crear materiales novedosos con propiedades específicas .
Safety and Hazards
Direcciones Futuras
Nalpha-Fmoc-D-histidine is a valuable resource for research in the post-genomic world . Its many beneficial attributes allow for very rapid and highly efficient synthesis of peptides, including ones of significant size and complexity . It’s also used in proteomics research , indicating its potential in the field of protein study and manipulation.
Mecanismo De Acción
Target of Action
The primary target of Fmoc-D-His is the amine group of amino acids . The Fmoc group is frequently used as a protecting group for amines, where it can be introduced by reacting the amine with fluorenylmethyloxycarbonyl chloride (Fmoc-Cl) .
Mode of Action
The Fmoc group acts as a protecting group for the amine group of amino acids during peptide synthesis . It is introduced by reacting the amine with Fmoc-Cl . The Fmoc group is base-labile, meaning it can be removed under basic conditions . Piperidine is usually preferred for Fmoc group removal as it forms a stable adduct with the dibenzofulvene byproduct, preventing it from reacting with the substrate .
Biochemical Pathways
The Fmoc group plays a crucial role in the chemical synthesis of peptides . It allows for the rapid and highly efficient synthesis of peptides, including ones of significant size and complexity .
Pharmacokinetics
The fmoc group’s stability to treatment with trifluoroacetic acid (tfa) and hydrogen bromide/acetic acid has been noted . This stability suggests that the Fmoc group may influence the ADME (Absorption, Distribution, Metabolism, and Excretion) properties of the compound.
Result of Action
The primary result of Fmoc-D-His’s action is the formation of protected peptides during peptide synthesis . The Fmoc group protects the amine group of amino acids, allowing for the efficient synthesis of complex peptides .
Action Environment
The action of Fmoc-D-His is influenced by the pH of the environment . The Fmoc group is base-labile, meaning it can be removed under basic conditions . Therefore, the pH of the environment can influence the efficacy and stability of Fmoc-D-His. Additionally, the Fmoc group is stable to treatment with TFA and hydrogen bromide/acetic acid, suggesting that it may also be stable under acidic conditions .
Análisis Bioquímico
Biochemical Properties
In biochemical reactions, FMOC-D-HIS-OH plays a crucial role as a building block in peptide synthesis . The Fmoc group in this compound can be introduced by reacting the amine with fluorenylmethyloxycarbonyl chloride (Fmoc-Cl) . This process is essential in the formation of peptides, as it provides protection against racemization and by-product formation .
Cellular Effects
The cellular effects of this compound are primarily related to its role in peptide synthesis. As a building block in this process, this compound contributes to the formation of peptides that can influence various cellular processes, including cell signaling pathways, gene expression, and cellular metabolism .
Molecular Mechanism
The molecular mechanism of this compound involves its role as a protecting group in peptide synthesis. The Fmoc group in this compound is base-labile, meaning it can be rapidly removed by a base . This property allows for the controlled assembly and disassembly of peptides during synthesis .
Temporal Effects in Laboratory Settings
In laboratory settings, the effects of this compound can change over time, particularly in relation to its stability and degradation. The Fmoc group in this compound is stable under certain conditions, which is crucial for its role in peptide synthesis . It can be rapidly removed by a base, such as piperidine .
Metabolic Pathways
This compound is involved in the metabolic pathway of peptide synthesis . In this process, this compound interacts with other amino acids, enzymes, and cofactors to form peptides .
Transport and Distribution
The transport and distribution of this compound within cells and tissues are closely tied to its role in peptide synthesis. As a building block in this process, this compound is transported to the site of peptide synthesis where it interacts with other molecules to form peptides .
Subcellular Localization
The subcellular localization of this compound is related to its role in peptide synthesis. During this process, this compound is typically localized at the site of peptide synthesis . The exact subcellular localization can vary depending on the specific cellular context and the other molecules involved in the synthesis process.
Propiedades
IUPAC Name |
(2R)-2-(9H-fluoren-9-ylmethoxycarbonylamino)-3-(1H-imidazol-5-yl)propanoic acid | |
---|---|---|
Source | PubChem | |
URL | https://pubchem.ncbi.nlm.nih.gov | |
Description | Data deposited in or computed by PubChem | |
InChI |
InChI=1S/C21H19N3O4/c25-20(26)19(9-13-10-22-12-23-13)24-21(27)28-11-18-16-7-3-1-5-14(16)15-6-2-4-8-17(15)18/h1-8,10,12,18-19H,9,11H2,(H,22,23)(H,24,27)(H,25,26)/t19-/m1/s1 | |
Source | PubChem | |
URL | https://pubchem.ncbi.nlm.nih.gov | |
Description | Data deposited in or computed by PubChem | |
InChI Key |
SIRPVCUJLVXZPW-LJQANCHMSA-N | |
Source | PubChem | |
URL | https://pubchem.ncbi.nlm.nih.gov | |
Description | Data deposited in or computed by PubChem | |
Canonical SMILES |
C1=CC=C2C(=C1)C(C3=CC=CC=C32)COC(=O)NC(CC4=CN=CN4)C(=O)O | |
Source | PubChem | |
URL | https://pubchem.ncbi.nlm.nih.gov | |
Description | Data deposited in or computed by PubChem | |
Isomeric SMILES |
C1=CC=C2C(=C1)C(C3=CC=CC=C32)COC(=O)N[C@H](CC4=CN=CN4)C(=O)O | |
Source | PubChem | |
URL | https://pubchem.ncbi.nlm.nih.gov | |
Description | Data deposited in or computed by PubChem | |
Molecular Formula |
C21H19N3O4 | |
Source | PubChem | |
URL | https://pubchem.ncbi.nlm.nih.gov | |
Description | Data deposited in or computed by PubChem | |
DSSTOX Substance ID |
DTXSID50427162 | |
Record name | Nalpha-Fmoc-D-histidine | |
Source | EPA DSSTox | |
URL | https://comptox.epa.gov/dashboard/DTXSID50427162 | |
Description | DSSTox provides a high quality public chemistry resource for supporting improved predictive toxicology. | |
Molecular Weight |
377.4 g/mol | |
Source | PubChem | |
URL | https://pubchem.ncbi.nlm.nih.gov | |
Description | Data deposited in or computed by PubChem | |
CAS RN |
157355-79-8 | |
Record name | Nalpha-Fmoc-D-histidine | |
Source | EPA DSSTox | |
URL | https://comptox.epa.gov/dashboard/DTXSID50427162 | |
Description | DSSTox provides a high quality public chemistry resource for supporting improved predictive toxicology. | |
Descargo de responsabilidad e información sobre productos de investigación in vitro
Tenga en cuenta que todos los artículos e información de productos presentados en BenchChem están destinados únicamente con fines informativos. Los productos disponibles para la compra en BenchChem están diseñados específicamente para estudios in vitro, que se realizan fuera de organismos vivos. Los estudios in vitro, derivados del término latino "in vidrio", involucran experimentos realizados en entornos de laboratorio controlados utilizando células o tejidos. Es importante tener en cuenta que estos productos no se clasifican como medicamentos y no han recibido la aprobación de la FDA para la prevención, tratamiento o cura de ninguna condición médica, dolencia o enfermedad. Debemos enfatizar que cualquier forma de introducción corporal de estos productos en humanos o animales está estrictamente prohibida por ley. Es esencial adherirse a estas pautas para garantizar el cumplimiento de los estándares legales y éticos en la investigación y experimentación.