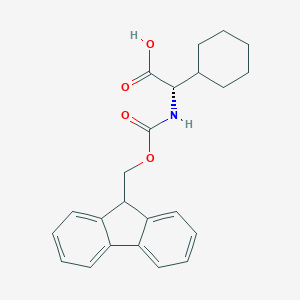
Fmoc-Chg-OH
Descripción general
Descripción
Aplicaciones Científicas De Investigación
Chemistry
Fmoc-Chg-OH is extensively used in the synthesis of peptides and proteins. Its stability and ease of removal make it an ideal protecting group for the amino terminus in SPPS .
Biology and Medicine
In biological and medical research, this compound is used to synthesize peptide-based hydrogels, which have applications in drug delivery, tissue engineering, and as scaffolds for cell culture .
Industry
In the pharmaceutical industry, this compound is used in the synthesis of therapeutic peptides and proteins. Its use in SPPS allows for the efficient production of high-purity peptides .
Mecanismo De Acción
Target of Action
Fmoc-Chg-OH, also known as Fluorenylmethyloxycarbonyl Cyclohexylglycine, is primarily used as a protecting group for amines in peptide synthesis . The primary target of this compound is the amine group of amino acids, which it protects during the synthesis process .
Mode of Action
The Fmoc group can be introduced by reacting the amine with fluorenylmethyloxycarbonyl chloride (Fmoc-Cl) . This reaction results in the formation of a carbamate, which serves as a protective group for the amine . The Fmoc group is base-labile, meaning it can be removed rapidly by a base . Piperidine is usually preferred for Fmoc group removal as it forms a stable adduct with the dibenzofulvene byproduct, preventing it from reacting with the substrate .
Biochemical Pathways
The use of this compound in peptide synthesis affects the biochemical pathway of peptide bond formation. The Fmoc group serves as a temporary protecting group for the N-terminus in Solid-Phase Peptide Synthesis (SPPS), allowing for the step-by-step assembly of the peptide chain .
Result of Action
The primary result of this compound’s action is the successful synthesis of peptides. By protecting the amine group during synthesis, this compound allows for the controlled formation of peptide bonds, enabling the creation of peptides of significant size and complexity .
Action Environment
The action of this compound is influenced by the chemical environment in which peptide synthesis occurs. For instance, the presence of a base is necessary for the removal of the Fmoc group . Additionally, the stability of this compound can be affected by factors such as temperature and pH.
Safety and Hazards
Fmoc-Chg-OH is harmful by inhalation, in contact with skin, and if swallowed . In case of skin contact, it is recommended to wash skin with copious amounts of water for at least 15 minutes while removing contaminated clothing and shoes . In case of eye contact, rinse with pure water for at least 15 minutes . If inhaled, remove to fresh air .
Análisis Bioquímico
Biochemical Properties
Fmoc-Chg-OH plays a role in biochemical reactions as a protecting group for amines . The Fmoc group can be introduced by reacting the amine with fluorenylmethyloxycarbonyl chloride (Fmoc-Cl) . The Fmoc group is used as a temporary protecting group for the N-terminus in solid-phase peptide synthesis .
Cellular Effects
The cellular effects of this compound are primarily related to its role in peptide synthesis. The Fmoc group allows for rapid and efficient synthesis of peptides, including those of significant size and complexity . This makes this compound an extremely valuable resource for research in the post-genomic world .
Molecular Mechanism
The molecular mechanism of this compound involves the protection of the N-terminus in peptide synthesis. The Fmoc group is cleaved by secondary amines such as piperidine . After the peptide has been assembled, it is removed from the resin by treatment with trifluoroacetic acid (TFA), while protecting groups on amino acid side chains are simultaneously removed .
Temporal Effects in Laboratory Settings
The temporal effects of this compound in laboratory settings are related to its stability and degradation during peptide synthesis. The Fmoc group is rapidly removed by base . Piperidine is usually preferred for Fmoc group removal as it forms a stable adduct with the dibenzofulvene byproduct, preventing it from reacting with the substrate .
Metabolic Pathways
The metabolic pathways involving this compound are primarily related to its role in peptide synthesis. The Fmoc group is part of the process of solid-phase peptide synthesis, which involves the step-by-step assembly of the peptide chain .
Transport and Distribution
The transport and distribution of this compound within cells and tissues are related to its role in peptide synthesis. In Fmoc solid-phase peptide synthesis, the peptide chain is assembled step by step, one amino acid at a time, while attached to an insoluble resin support .
Subcellular Localization
The subcellular localization of this compound is related to its role in peptide synthesis. As part of the Fmoc group, this compound is involved in the process of solid-phase peptide synthesis, which occurs in the cytoplasm of the cell .
Métodos De Preparación
Synthetic Routes and Reaction Conditions
Fmoc-Chg-OH can be synthesized through several methods. One common approach involves the protection of the amino group of cyclohexylglycine with the Fmoc group. This can be achieved by reacting cyclohexylglycine with fluorenylmethyloxycarbonyl chloride (Fmoc-Cl) in the presence of a base such as sodium bicarbonate in an aqueous dioxane solution . Another method involves the use of 9-fluorenylmethylsuccinimidyl carbonate (Fmoc-OSu), which is obtained by reacting Fmoc-Cl with the dicyclohexylammonium salt of N-hydroxysuccinimide .
Industrial Production Methods
Industrial production of this compound typically involves large-scale solid-phase peptide synthesis (SPPS). The process includes the stepwise addition of amino acids to a growing peptide chain anchored to an insoluble resin. The Fmoc group is used as a temporary protecting group for the amino terminus, which is removed by treatment with piperidine .
Análisis De Reacciones Químicas
Types of Reactions
Fmoc-Chg-OH undergoes several types of chemical reactions, including:
Deprotection: The Fmoc group can be removed under basic conditions, typically using piperidine in dimethylformamide (DMF).
Coupling Reactions: The carboxyl group of this compound can be activated and coupled with other amino acids or peptides using reagents such as carbodiimides (e.g., DCC or EDC) and additives like HOBt or HOAt.
Common Reagents and Conditions
Deprotection: 20% piperidine in DMF is commonly used for Fmoc deprotection.
Major Products Formed
The major products formed from these reactions include deprotected amino acids or peptides ready for further elongation or modification in peptide synthesis .
Comparación Con Compuestos Similares
Similar Compounds
Fmoc-Gly-OH: Fmoc-protected glycine.
Fmoc-Ala-OH: Fmoc-protected alanine.
Fmoc-Val-OH: Fmoc-protected valine.
Uniqueness
Fmoc-Chg-OH is unique due to the presence of the cyclohexyl group, which imparts distinct steric and hydrophobic properties. This makes it particularly useful in the synthesis of peptides that require these specific characteristics .
Propiedades
IUPAC Name |
(2S)-2-cyclohexyl-2-(9H-fluoren-9-ylmethoxycarbonylamino)acetic acid | |
---|---|---|
Source | PubChem | |
URL | https://pubchem.ncbi.nlm.nih.gov | |
Description | Data deposited in or computed by PubChem | |
InChI |
InChI=1S/C23H25NO4/c25-22(26)21(15-8-2-1-3-9-15)24-23(27)28-14-20-18-12-6-4-10-16(18)17-11-5-7-13-19(17)20/h4-7,10-13,15,20-21H,1-3,8-9,14H2,(H,24,27)(H,25,26)/t21-/m0/s1 | |
Source | PubChem | |
URL | https://pubchem.ncbi.nlm.nih.gov | |
Description | Data deposited in or computed by PubChem | |
InChI Key |
BWQQGHPODCJZDB-NRFANRHFSA-N | |
Source | PubChem | |
URL | https://pubchem.ncbi.nlm.nih.gov | |
Description | Data deposited in or computed by PubChem | |
Canonical SMILES |
C1CCC(CC1)C(C(=O)O)NC(=O)OCC2C3=CC=CC=C3C4=CC=CC=C24 | |
Source | PubChem | |
URL | https://pubchem.ncbi.nlm.nih.gov | |
Description | Data deposited in or computed by PubChem | |
Isomeric SMILES |
C1CCC(CC1)[C@@H](C(=O)O)NC(=O)OCC2C3=CC=CC=C3C4=CC=CC=C24 | |
Source | PubChem | |
URL | https://pubchem.ncbi.nlm.nih.gov | |
Description | Data deposited in or computed by PubChem | |
Molecular Formula |
C23H25NO4 | |
Source | PubChem | |
URL | https://pubchem.ncbi.nlm.nih.gov | |
Description | Data deposited in or computed by PubChem | |
DSSTOX Substance ID |
DTXSID70426273 | |
Record name | Fmoc-Chg-OH | |
Source | EPA DSSTox | |
URL | https://comptox.epa.gov/dashboard/DTXSID70426273 | |
Description | DSSTox provides a high quality public chemistry resource for supporting improved predictive toxicology. | |
Molecular Weight |
379.4 g/mol | |
Source | PubChem | |
URL | https://pubchem.ncbi.nlm.nih.gov | |
Description | Data deposited in or computed by PubChem | |
CAS No. |
161321-36-4 | |
Record name | Fmoc-Chg-OH | |
Source | EPA DSSTox | |
URL | https://comptox.epa.gov/dashboard/DTXSID70426273 | |
Description | DSSTox provides a high quality public chemistry resource for supporting improved predictive toxicology. | |
Retrosynthesis Analysis
AI-Powered Synthesis Planning: Our tool employs the Template_relevance Pistachio, Template_relevance Bkms_metabolic, Template_relevance Pistachio_ringbreaker, Template_relevance Reaxys, Template_relevance Reaxys_biocatalysis model, leveraging a vast database of chemical reactions to predict feasible synthetic routes.
One-Step Synthesis Focus: Specifically designed for one-step synthesis, it provides concise and direct routes for your target compounds, streamlining the synthesis process.
Accurate Predictions: Utilizing the extensive PISTACHIO, BKMS_METABOLIC, PISTACHIO_RINGBREAKER, REAXYS, REAXYS_BIOCATALYSIS database, our tool offers high-accuracy predictions, reflecting the latest in chemical research and data.
Strategy Settings
Precursor scoring | Relevance Heuristic |
---|---|
Min. plausibility | 0.01 |
Model | Template_relevance |
Template Set | Pistachio/Bkms_metabolic/Pistachio_ringbreaker/Reaxys/Reaxys_biocatalysis |
Top-N result to add to graph | 6 |
Feasible Synthetic Routes
Descargo de responsabilidad e información sobre productos de investigación in vitro
Tenga en cuenta que todos los artículos e información de productos presentados en BenchChem están destinados únicamente con fines informativos. Los productos disponibles para la compra en BenchChem están diseñados específicamente para estudios in vitro, que se realizan fuera de organismos vivos. Los estudios in vitro, derivados del término latino "in vidrio", involucran experimentos realizados en entornos de laboratorio controlados utilizando células o tejidos. Es importante tener en cuenta que estos productos no se clasifican como medicamentos y no han recibido la aprobación de la FDA para la prevención, tratamiento o cura de ninguna condición médica, dolencia o enfermedad. Debemos enfatizar que cualquier forma de introducción corporal de estos productos en humanos o animales está estrictamente prohibida por ley. Es esencial adherirse a estas pautas para garantizar el cumplimiento de los estándares legales y éticos en la investigación y experimentación.