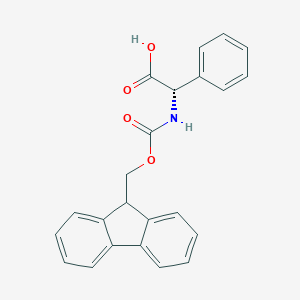
Fmoc-Phg-OH
Descripción general
Descripción
Fmoc-L-alpha-phenylglycine, commonly referred to as Fmoc-Phg-OH, is a derivative of phenylglycine. It is widely used in the field of peptide synthesis, particularly in solid-phase peptide synthesis (SPPS). The compound is characterized by the presence of a fluorenylmethyloxycarbonyl (Fmoc) protecting group, which is base-labile and can be easily removed during peptide synthesis .
Métodos De Preparación
Synthetic Routes and Reaction Conditions
Fmoc-Phg-OH is typically synthesized through the reaction of phenylglycine with fluorenylmethyloxycarbonyl chloride (Fmoc-Cl) in the presence of a base such as sodium bicarbonate. The reaction proceeds via nucleophilic attack of the amine group on phenylglycine to the carbonyl carbon of Fmoc-Cl, resulting in the formation of this compound .
Industrial Production Methods
In industrial settings, the production of this compound often involves solid-phase peptide synthesis (SPPS). This method allows for the efficient and scalable production of peptides and their derivatives. The process involves anchoring the amino acid to a solid support and sequentially adding protected amino acids to build the desired peptide chain .
Análisis De Reacciones Químicas
Types of Reactions
Fmoc-Phg-OH undergoes several types of chemical reactions, including:
Oxidation: The phenyl group can be oxidized under specific conditions.
Reduction: The carbonyl group can be reduced to form alcohols.
Substitution: The Fmoc group can be substituted with other protecting groups or functional groups.
Common Reagents and Conditions
Oxidation: Common oxidizing agents include potassium permanganate and chromium trioxide.
Reduction: Reducing agents such as lithium aluminum hydride and sodium borohydride are commonly used.
Substitution: The Fmoc group is typically removed using piperidine in N,N-dimethylformamide (DMF) solution.
Major Products Formed
The major products formed from these reactions include deprotected phenylglycine, oxidized derivatives, and reduced alcohols.
Aplicaciones Científicas De Investigación
Fmoc-Phg-OH has a wide range of applications in scientific research:
Chemistry: It is used in the synthesis of peptides and peptidomimetics.
Biology: It serves as a building block for the synthesis of biologically active peptides.
Medicine: It is used in the development of peptide-based drugs and therapeutic agents.
Industry: It is employed in the production of hydrogels and other biomaterials for tissue engineering and drug delivery
Mecanismo De Acción
The mechanism of action of Fmoc-Phg-OH primarily involves its role as a protecting group in peptide synthesis. The Fmoc group protects the amine group of phenylglycine during the synthesis process, preventing unwanted side reactions. The Fmoc group is removed by base treatment, typically with piperidine, which forms a stable adduct with the dibenzofulvene byproduct, thus preventing it from reacting with the substrate .
Comparación Con Compuestos Similares
Similar Compounds
Fmoc-L-phenylalanine: Similar in structure but with an additional methylene group.
Fmoc-D-phenylglycine: The D-enantiomer of Fmoc-Phg-OH.
Fmoc-propargyl-glycine: Contains a propargyl group instead of a phenyl group
Uniqueness
This compound is unique due to its specific structure, which allows for the incorporation of phenylglycine residues into peptides. This can impart unique properties to the resulting peptides, such as increased stability and bioactivity.
Actividad Biológica
Fmoc-Phg-OH, or Fmoc-L-alpha-phenylglycine, is a significant compound in the field of peptide synthesis and has garnered attention for its biological activity and applications in various scientific domains. This article delves into its biochemical properties, cellular effects, molecular mechanisms, and applications in research and medicine.
Overview of this compound
This compound is a derivative of phenylglycine, primarily utilized as a protecting group for amines during solid-phase peptide synthesis (SPPS). The fluorenylmethyloxycarbonyl (Fmoc) group provides stability and facilitates the selective protection of the amino group, allowing for the efficient assembly of peptides without interference from other functional groups.
This compound exhibits several biochemical properties that contribute to its functionality in peptide synthesis:
- Protecting Group : It effectively shields the N-terminus of peptides during synthesis, which is crucial for maintaining the integrity of the growing peptide chain.
- Fluorescence : The fluorenyl group is highly fluorescent, enabling real-time monitoring of peptide synthesis through techniques like reversed-phase high-performance liquid chromatography (HPLC).
- Solubility : It demonstrates good solubility in organic solvents such as DMF, which is essential for its application in SPPS .
Cellular Effects
The biological activity of this compound is primarily linked to the peptides synthesized using this compound. These peptides can modulate various cellular processes:
- Cell Signaling : Peptides derived from this compound can influence signaling pathways, potentially affecting cell proliferation, differentiation, and apoptosis.
- Gene Expression : Certain peptides may regulate gene expression by interacting with transcription factors or other regulatory proteins.
- Metabolic Pathways : The synthesized peptides can participate in metabolic processes, influencing cellular metabolism and energy production .
Molecular Mechanisms
The molecular mechanisms underlying the activity of this compound involve its role in peptide synthesis:
- Peptide Bond Formation : this compound contributes to the formation of peptide bonds, a fundamental process in protein synthesis.
- Deprotection Reactions : The Fmoc group can be removed using piperidine in DMF, allowing for the release of free amines that can participate in further reactions .
- Self-Assembly : The resulting peptides can undergo self-assembly due to hydrophobic interactions and π-π stacking, leading to the formation of supramolecular structures with potential biological functions .
Research Applications
This compound has diverse applications across various fields:
Case Studies and Research Findings
Recent studies have highlighted the utility of this compound in synthesizing antimicrobial peptides (AMPs) that show promise against multi-drug-resistant pathogens. For instance, research demonstrated that specific AMPs synthesized using Fmoc-protected amino acids exhibited significant antimicrobial activity, showcasing their potential as novel therapeutic agents .
In another study focused on hydrogels formed from Fmoc-protected dipeptides, researchers found that these materials could encapsulate and release model drugs effectively, indicating their potential use in drug delivery systems .
Propiedades
IUPAC Name |
(2S)-2-(9H-fluoren-9-ylmethoxycarbonylamino)-2-phenylacetic acid | |
---|---|---|
Source | PubChem | |
URL | https://pubchem.ncbi.nlm.nih.gov | |
Description | Data deposited in or computed by PubChem | |
InChI |
InChI=1S/C23H19NO4/c25-22(26)21(15-8-2-1-3-9-15)24-23(27)28-14-20-18-12-6-4-10-16(18)17-11-5-7-13-19(17)20/h1-13,20-21H,14H2,(H,24,27)(H,25,26)/t21-/m0/s1 | |
Source | PubChem | |
URL | https://pubchem.ncbi.nlm.nih.gov | |
Description | Data deposited in or computed by PubChem | |
InChI Key |
PCJHOCNJLMFYCV-NRFANRHFSA-N | |
Source | PubChem | |
URL | https://pubchem.ncbi.nlm.nih.gov | |
Description | Data deposited in or computed by PubChem | |
Canonical SMILES |
C1=CC=C(C=C1)C(C(=O)O)NC(=O)OCC2C3=CC=CC=C3C4=CC=CC=C24 | |
Source | PubChem | |
URL | https://pubchem.ncbi.nlm.nih.gov | |
Description | Data deposited in or computed by PubChem | |
Isomeric SMILES |
C1=CC=C(C=C1)[C@@H](C(=O)O)NC(=O)OCC2C3=CC=CC=C3C4=CC=CC=C24 | |
Source | PubChem | |
URL | https://pubchem.ncbi.nlm.nih.gov | |
Description | Data deposited in or computed by PubChem | |
Molecular Formula |
C23H19NO4 | |
Source | PubChem | |
URL | https://pubchem.ncbi.nlm.nih.gov | |
Description | Data deposited in or computed by PubChem | |
DSSTOX Substance ID |
DTXSID80428480 | |
Record name | Fmoc-Phg-OH | |
Source | EPA DSSTox | |
URL | https://comptox.epa.gov/dashboard/DTXSID80428480 | |
Description | DSSTox provides a high quality public chemistry resource for supporting improved predictive toxicology. | |
Molecular Weight |
373.4 g/mol | |
Source | PubChem | |
URL | https://pubchem.ncbi.nlm.nih.gov | |
Description | Data deposited in or computed by PubChem | |
CAS No. |
102410-65-1 | |
Record name | Fmoc-Phg-OH | |
Source | EPA DSSTox | |
URL | https://comptox.epa.gov/dashboard/DTXSID80428480 | |
Description | DSSTox provides a high quality public chemistry resource for supporting improved predictive toxicology. | |
Record name | (2S)-2-{[(9H-fluoren-9-ylmethoxy)carbonyl]amino}-2-phenylacetic acid | |
Source | European Chemicals Agency (ECHA) | |
URL | https://echa.europa.eu/information-on-chemicals | |
Description | The European Chemicals Agency (ECHA) is an agency of the European Union which is the driving force among regulatory authorities in implementing the EU's groundbreaking chemicals legislation for the benefit of human health and the environment as well as for innovation and competitiveness. | |
Explanation | Use of the information, documents and data from the ECHA website is subject to the terms and conditions of this Legal Notice, and subject to other binding limitations provided for under applicable law, the information, documents and data made available on the ECHA website may be reproduced, distributed and/or used, totally or in part, for non-commercial purposes provided that ECHA is acknowledged as the source: "Source: European Chemicals Agency, http://echa.europa.eu/". Such acknowledgement must be included in each copy of the material. ECHA permits and encourages organisations and individuals to create links to the ECHA website under the following cumulative conditions: Links can only be made to webpages that provide a link to the Legal Notice page. | |
Retrosynthesis Analysis
AI-Powered Synthesis Planning: Our tool employs the Template_relevance Pistachio, Template_relevance Bkms_metabolic, Template_relevance Pistachio_ringbreaker, Template_relevance Reaxys, Template_relevance Reaxys_biocatalysis model, leveraging a vast database of chemical reactions to predict feasible synthetic routes.
One-Step Synthesis Focus: Specifically designed for one-step synthesis, it provides concise and direct routes for your target compounds, streamlining the synthesis process.
Accurate Predictions: Utilizing the extensive PISTACHIO, BKMS_METABOLIC, PISTACHIO_RINGBREAKER, REAXYS, REAXYS_BIOCATALYSIS database, our tool offers high-accuracy predictions, reflecting the latest in chemical research and data.
Strategy Settings
Precursor scoring | Relevance Heuristic |
---|---|
Min. plausibility | 0.01 |
Model | Template_relevance |
Template Set | Pistachio/Bkms_metabolic/Pistachio_ringbreaker/Reaxys/Reaxys_biocatalysis |
Top-N result to add to graph | 6 |
Feasible Synthetic Routes
Q1: What is the main challenge encountered when incorporating Fmoc-Phenylglycine (Fmoc-Phg-OH) into peptides using standard solid-phase peptide synthesis (SPPS) protocols?
A: Fmoc-Phenylglycine (this compound) is highly prone to racemization during standard SPPS, particularly during the Fmoc deprotection step []. This means that the desired L-enantiomer can partially convert to the D-enantiomer, leading to a mixture of diastereomers in the final peptide product. This is problematic because even small amounts of D-amino acids can significantly impact a peptide's biological activity and conformational properties.
Q2: The research mentions that Fmoc-Phenylalanine (Fmoc-Phe-OH) doesn't exhibit this racemization problem. What makes Fmoc-Phenylglycine (this compound) more susceptible?
A: The increased susceptibility of Fmoc-Phenylglycine (this compound) to racemization compared to Fmoc-Phenylalanine (Fmoc-Phe-OH) is due to the presence of an alpha-hydrogen in phenylglycine. This alpha-hydrogen is more acidic due to the electron-withdrawing nature of the phenyl ring, making it easier to abstract by bases. This abstraction generates a carbanion intermediate, which is planar and can be reprotonated from either side, leading to racemization [].
Descargo de responsabilidad e información sobre productos de investigación in vitro
Tenga en cuenta que todos los artículos e información de productos presentados en BenchChem están destinados únicamente con fines informativos. Los productos disponibles para la compra en BenchChem están diseñados específicamente para estudios in vitro, que se realizan fuera de organismos vivos. Los estudios in vitro, derivados del término latino "in vidrio", involucran experimentos realizados en entornos de laboratorio controlados utilizando células o tejidos. Es importante tener en cuenta que estos productos no se clasifican como medicamentos y no han recibido la aprobación de la FDA para la prevención, tratamiento o cura de ninguna condición médica, dolencia o enfermedad. Debemos enfatizar que cualquier forma de introducción corporal de estos productos en humanos o animales está estrictamente prohibida por ley. Es esencial adherirse a estas pautas para garantizar el cumplimiento de los estándares legales y éticos en la investigación y experimentación.