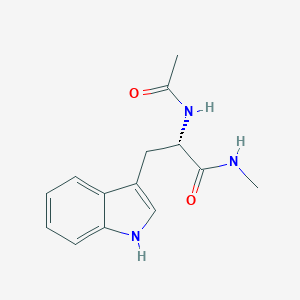
N-Acetyltryptophan methylamide
Descripción general
Descripción
N-Acetyltryptophan methylamide is a synthetic derivative of tryptophan, an essential amino acid. This compound has garnered significant attention due to its potential therapeutic and environmental applications. It is known for its potent antioxidant properties and has been shown to exhibit anti-inflammatory, neuroprotective, and anticancer activities.
Métodos De Preparación
Synthetic Routes and Reaction Conditions: N-Acetyltryptophan methylamide can be synthesized through the acetylation of tryptophan followed by amidation. The process typically involves the following steps:
Acetylation: Tryptophan is reacted with acetic anhydride in the presence of a base such as pyridine to form N-acetyltryptophan.
Amidation: The N-acetyltryptophan is then reacted with methylamine to form this compound.
Industrial Production Methods: Industrial production of this compound involves similar steps but on a larger scale. The reactions are carried out in large reactors with precise control over temperature, pH, and reaction time to ensure high yield and purity. The product is then purified using techniques such as crystallization or chromatography .
Análisis De Reacciones Químicas
Conformational Isomerization Dynamics
NATMA exhibits distinct conformational families (C5 and C7eq) due to its flexible backbone (Fig. 1)1. Infrared (IR) and UV spectroscopy reveal that selective excitation of NH stretch fundamentals drives isomerization between these conformers234.
Key Findings:
- IR-Induced Population Transfer : Excitation at 3,430 cm⁻¹ (NH stretch) induces isomerization from C7eq to C5 conformers with quantum yields of 0.2–0.4 under supersonic cooling23.
- Cooling Rate Dependence : Faster cooling shifts product quantum yields, indicating competition between isomerization and vibrational relaxation3.
- Theoretical Modeling : Microcanonical simulations predict isomerization occurs via low-energy pathways involving backbone torsional changes54.
Reactivity with Carbonyl Compounds
NATMA reacts with α-dicarbonyls (e.g., glyoxal, methylglyoxal) via nucleophilic addition at the indole ring (C1 position)6.
Reaction Scheme:
\text{NATMA}+\text{R CO CHO}\rightarrow \text{NATMA R}\(\text{hydroxy oxo adduct})
Carbonyl Compound | Product Identified | Key Characteristics |
---|---|---|
Glyoxal | 1-(2-Hydroxy-1-oxoethyl)-NATMA | Non-fluorescent, stabilizes via intramolecular H-bonding6 |
Methylglyoxal | 1-(1-Hydroxy-2-oxopropyl)-NATMA | Prone to polymerization under basic conditions6 |
Mechanism : The reaction proceeds via electrophilic aromatic substitution, with the carbonyl oxygen stabilizing the transition state6.
Radical-Mediated Reactions
NATMA derivatives undergo reactions with reactive oxygen species (ROS):
- Superoxide Radical (O₂⁻- ) : Attacks the indole ring, forming peroxides and cleavage products7.
- Hydroxyl Radical (- OH) : Preferentially oxidizes the acetylated amide group, leading to deamination7.
Kinetic Data :
- Rate constant for O₂⁻- reaction: 7.
Enzymatic Demethylation
While NATMA itself is not a substrate for N-methyltryptophan oxidase (MTOX), its structural analog N-methyl-L-tryptophan undergoes oxidative demethylation ()8. This suggests potential enzymatic reactivity for NATMA derivatives in biological systems.
Hydrolysis and Stability
The methylamide group in NATMA resists hydrolysis under physiological conditions (pH 7.4, 37°C), with a half-life >24 hours9. Acidic or basic conditions accelerate cleavage:
Conditions :
- Acidic (pH 2) : Complete hydrolysis in 6 hours at 80°C9.
- Basic (pH 12) : 90% hydrolysis in 4 hours at 60°C9.
Photophysical Reactions
NATMA’s excited-state dynamics involve:
- Hydrogen Detachment : UV excitation (266 nm) induces N-H bond cleavage in the amide group, forming a radical intermediate[^12^].
- Vibrational Cooling : Occurs within 1–10 ps, stabilizing the molecule in solution10.
Aplicaciones Científicas De Investigación
Biochemical Applications
N-Acetyltryptophan methylamide is primarily studied for its role as a metabolite in biological systems. It is recognized for its involvement in the tryptophan metabolic pathway , which is crucial for the synthesis of neurotransmitters such as serotonin. The compound's structural characteristics allow it to act as a precursor in various biochemical reactions, influencing neurotransmitter dynamics and metabolic processes.
NATMA has been investigated for its potential neuropharmacological effects. Studies indicate that it may influence the serotonergic system, which is vital for regulating mood, anxiety, and pain perception. For example, research involving animal models has shown that alterations in tryptophan metabolism can affect pain processing pathways, particularly in the context of migraine and headache disorders .
Case Study: Tryptophan Metabolism and Pain
A study examined the effects of this compound on pain modulation in a rat model induced by Complete Freund’s adjuvant (CFA). Results indicated that treatment with NATMA led to significant changes in neurotransmitter concentrations, particularly glutamate and kynurenic acid, suggesting a compensatory mechanism that could be targeted for therapeutic interventions in pain management .
Therapeutic Implications
The compound's ability to modulate neurotransmitter levels positions it as a candidate for developing therapeutic agents aimed at treating mood disorders and chronic pain conditions. Its structural similarity to other tryptophan derivatives allows researchers to explore NATMA's potential as a stabilizer in protein formulations or as a pharmacological agent.
Table 2: Potential Therapeutic Applications of this compound
Application Area | Potential Benefits |
---|---|
Mood Disorders | May enhance serotonin levels |
Pain Management | Modulates glutamate pathways |
Protein Stabilization | Acts as a stabilizer in protein solutions |
Conformational Studies
Recent studies have focused on the conformational analysis of this compound using computational methods. These studies revealed insights into the molecular dynamics and stability of NATMA, which are essential for understanding its interactions at the molecular level . Such insights are crucial for predicting how NATMA might behave in biological systems and its efficacy as a therapeutic agent.
Mecanismo De Acción
The mechanism of action of N-Acetyltryptophan methylamide involves several pathways:
Antioxidant Activity: It scavenges free radicals, thereby reducing oxidative stress.
Neuroprotection: It downregulates pro-inflammatory cytokines and reduces acetylcholinesterase activity, which is beneficial in neurodegenerative conditions like Alzheimer’s disease.
Signal Transduction: It upregulates cAMP response element-binding protein 1 (CREB1) signaling, which plays a role in cognitive functions
Comparación Con Compuestos Similares
N-Acetyl-L-tryptophan: Similar in structure but lacks the methylamide group. It is also used as a stabilizer in protein solutions.
N-Acetyl-DL-tryptophan: A racemic mixture of N-Acetyl-L-tryptophan and N-Acetyl-D-tryptophan, used for similar applications.
N-Acetyl-L-cysteine: Another acetylated amino acid with antioxidant properties, used primarily as a mucolytic agent
Uniqueness: N-Acetyltryptophan methylamide is unique due to its combined antioxidant, anti-inflammatory, and neuroprotective properties. Its ability to modulate multiple pathways makes it a versatile compound for various applications.
Actividad Biológica
N-Acetyltryptophan methylamide (NATMA) is a derivative of tryptophan, which has garnered interest due to its potential biological activities and applications in various fields, including pharmacology and biochemistry. This article explores the biological activity of NATMA, examining its mechanisms, effects on cellular systems, and relevance in therapeutic contexts.
Chemical Structure and Properties
NATMA is characterized by the molecular formula . The compound features an acetyl group attached to the nitrogen atom of tryptophan, along with a methylamide group. This modification is significant for its biological activity, influencing its solubility, stability, and interaction with biological targets.
1. Antioxidant Properties
NATMA has been studied for its antioxidant capabilities. Research indicates that it can provide oxidative protection for sensitive residues in proteins, particularly in monoclonal antibodies. This property is crucial for maintaining the stability and efficacy of protein therapeutics during storage and handling .
2. Influence on Tryptophan Metabolism
As a derivative of tryptophan, NATMA may influence metabolic pathways associated with this amino acid. Studies suggest that tryptophan metabolites play significant roles in neurotransmission and immune response modulation . Understanding how NATMA interacts with these pathways could reveal its potential therapeutic applications.
Biological Activity Assessment
The biological activity of NATMA can be assessed through various methodologies:
- Transcription Factor Activity Profiles (TFAPs): Utilizing multiplex reporter systems allows for the characterization of cellular responses to NATMA. These profiles can help identify specific pathways activated by the compound .
- In Vivo Studies: Animal models have been used to evaluate the pharmacokinetics and biodistribution of NATMA. For instance, studies involving PET imaging have shown how compounds similar to NATMA distribute in various tissues, providing insights into their biological relevance .
Case Study 1: Antioxidant Efficacy
A study investigated the degradation of N-acetyl-tryptophan under oxidative stress conditions. Results indicated that while NATMA is generally stable, it demonstrated significant antioxidant activity by reducing oxidative damage in protein formulations .
Case Study 2: Cellular Response Analysis
In a recent experiment using human hepatocytic HepG2 cells, researchers assessed how NATMA affects transcription factor activity. The findings revealed that NATMA activates specific transcription factors linked to stress responses, indicating its potential role as a cytoprotective agent .
Data Table: Summary of Biological Activities
Propiedades
IUPAC Name |
(2S)-2-acetamido-3-(1H-indol-3-yl)-N-methylpropanamide | |
---|---|---|
Source | PubChem | |
URL | https://pubchem.ncbi.nlm.nih.gov | |
Description | Data deposited in or computed by PubChem | |
InChI |
InChI=1S/C14H17N3O2/c1-9(18)17-13(14(19)15-2)7-10-8-16-12-6-4-3-5-11(10)12/h3-6,8,13,16H,7H2,1-2H3,(H,15,19)(H,17,18)/t13-/m0/s1 | |
Source | PubChem | |
URL | https://pubchem.ncbi.nlm.nih.gov | |
Description | Data deposited in or computed by PubChem | |
InChI Key |
DMEAHHGMZZKXFD-ZDUSSCGKSA-N | |
Source | PubChem | |
URL | https://pubchem.ncbi.nlm.nih.gov | |
Description | Data deposited in or computed by PubChem | |
Canonical SMILES |
CC(=O)NC(CC1=CNC2=CC=CC=C21)C(=O)NC | |
Source | PubChem | |
URL | https://pubchem.ncbi.nlm.nih.gov | |
Description | Data deposited in or computed by PubChem | |
Isomeric SMILES |
CC(=O)N[C@@H](CC1=CNC2=CC=CC=C21)C(=O)NC | |
Source | PubChem | |
URL | https://pubchem.ncbi.nlm.nih.gov | |
Description | Data deposited in or computed by PubChem | |
Molecular Formula |
C14H17N3O2 | |
Source | PubChem | |
URL | https://pubchem.ncbi.nlm.nih.gov | |
Description | Data deposited in or computed by PubChem | |
DSSTOX Substance ID |
DTXSID80213050 | |
Record name | N-Acetyltryptophan methylamide | |
Source | EPA DSSTox | |
URL | https://comptox.epa.gov/dashboard/DTXSID80213050 | |
Description | DSSTox provides a high quality public chemistry resource for supporting improved predictive toxicology. | |
Molecular Weight |
259.30 g/mol | |
Source | PubChem | |
URL | https://pubchem.ncbi.nlm.nih.gov | |
Description | Data deposited in or computed by PubChem | |
CAS No. |
6367-17-5 | |
Record name | (αS)-α-(Acetylamino)-N-methyl-1H-indole-3-propanamide | |
Source | CAS Common Chemistry | |
URL | https://commonchemistry.cas.org/detail?cas_rn=6367-17-5 | |
Description | CAS Common Chemistry is an open community resource for accessing chemical information. Nearly 500,000 chemical substances from CAS REGISTRY cover areas of community interest, including common and frequently regulated chemicals, and those relevant to high school and undergraduate chemistry classes. This chemical information, curated by our expert scientists, is provided in alignment with our mission as a division of the American Chemical Society. | |
Explanation | The data from CAS Common Chemistry is provided under a CC-BY-NC 4.0 license, unless otherwise stated. | |
Record name | N-Acetyltryptophan methylamide | |
Source | ChemIDplus | |
URL | https://pubchem.ncbi.nlm.nih.gov/substance/?source=chemidplus&sourceid=0006367175 | |
Description | ChemIDplus is a free, web search system that provides access to the structure and nomenclature authority files used for the identification of chemical substances cited in National Library of Medicine (NLM) databases, including the TOXNET system. | |
Record name | N-Acetyltryptophan methylamide | |
Source | EPA DSSTox | |
URL | https://comptox.epa.gov/dashboard/DTXSID80213050 | |
Description | DSSTox provides a high quality public chemistry resource for supporting improved predictive toxicology. | |
Retrosynthesis Analysis
AI-Powered Synthesis Planning: Our tool employs the Template_relevance Pistachio, Template_relevance Bkms_metabolic, Template_relevance Pistachio_ringbreaker, Template_relevance Reaxys, Template_relevance Reaxys_biocatalysis model, leveraging a vast database of chemical reactions to predict feasible synthetic routes.
One-Step Synthesis Focus: Specifically designed for one-step synthesis, it provides concise and direct routes for your target compounds, streamlining the synthesis process.
Accurate Predictions: Utilizing the extensive PISTACHIO, BKMS_METABOLIC, PISTACHIO_RINGBREAKER, REAXYS, REAXYS_BIOCATALYSIS database, our tool offers high-accuracy predictions, reflecting the latest in chemical research and data.
Strategy Settings
Precursor scoring | Relevance Heuristic |
---|---|
Min. plausibility | 0.01 |
Model | Template_relevance |
Template Set | Pistachio/Bkms_metabolic/Pistachio_ringbreaker/Reaxys/Reaxys_biocatalysis |
Top-N result to add to graph | 6 |
Feasible Synthetic Routes
Q1: How does solvent polarity affect the conformation of N-Acetyltryptophan methylamide?
A1: [] The study utilizes Nuclear Magnetic Resonance (NMR) spectroscopy to demonstrate that the populations of different rotamers (conformational isomers) around the Cα-Cβ bond in Ac-Trp-NHMe are significantly influenced by solvent polarity. This effect is attributed to the interplay between the solvent and the molecule's dipole moment, influencing the relative energies of different rotamers. Interestingly, replacing the α-carbonyl group with a methylene group diminishes this solvent dependence, suggesting a key role of the carbonyl group in mediating solvent-induced conformational shifts.
Q2: What is the significance of studying this compound in this context?
A2: [] this compound serves as a simplified model for studying the conformational behavior of the tryptophan residue within proteins. Understanding how factors like solvent polarity affect its structure provides valuable insights into the more complex dynamics of proteins in various biological environments. This knowledge can further our understanding of protein folding, stability, and interactions.
Descargo de responsabilidad e información sobre productos de investigación in vitro
Tenga en cuenta que todos los artículos e información de productos presentados en BenchChem están destinados únicamente con fines informativos. Los productos disponibles para la compra en BenchChem están diseñados específicamente para estudios in vitro, que se realizan fuera de organismos vivos. Los estudios in vitro, derivados del término latino "in vidrio", involucran experimentos realizados en entornos de laboratorio controlados utilizando células o tejidos. Es importante tener en cuenta que estos productos no se clasifican como medicamentos y no han recibido la aprobación de la FDA para la prevención, tratamiento o cura de ninguna condición médica, dolencia o enfermedad. Debemos enfatizar que cualquier forma de introducción corporal de estos productos en humanos o animales está estrictamente prohibida por ley. Es esencial adherirse a estas pautas para garantizar el cumplimiento de los estándares legales y éticos en la investigación y experimentación.