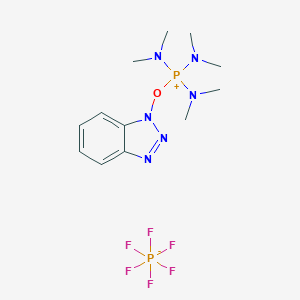
Reactivo BOP
Descripción general
Descripción
Benzotriazol-1-yloxytris(dimethylamino)phosphonium hexafluorophosphate is a chemical compound widely used in organic synthesis, particularly in peptide coupling reactions. It is known for its efficiency in forming amide bonds between carboxylic acids and amines, making it a valuable reagent in the field of peptide synthesis .
Aplicaciones Científicas De Investigación
Benzotriazol-1-yloxytris(dimethylamino)phosphonium hexafluorophosphate has a wide range of applications in scientific research:
Mecanismo De Acción
The BOP reagent, also known as Tri(dimethylamino)benzotriazol-1-yloxyphosphonium hexafluorophosphate, Castro’s Reagent, BOP hexafluorophosphate, or ((1H-Benzo[d][1,2,3]triazol-1-yl)oxy)tris(dimethylamino)phosphonium hexafluorophosphate(V), is a commonly used compound in the field of biochemistry .
Target of Action
The primary targets of the BOP reagent are peptides and carboxylic acids . It is used in the synthesis of peptides and the reduction of carboxylic acids .
Mode of Action
In peptide coupling, the BOP reagent works well because it forms reactive intermediates which allow for the amines to bond together with little energy loss . In the reduction of carboxylic acids, using the BOP reagent with NaBH4 resulted in high percent yields .
Biochemical Pathways
The BOP reagent is involved in the peptide synthesis pathway and the carboxylic acid reduction pathway . It plays a crucial role in these pathways by facilitating the formation of peptide bonds and the reduction of carboxylic acids to alcohols .
Pharmacokinetics
It is known that the bop reagent is partially soluble in cold water and decomposes upon reaction .
Action Environment
The BOP reagent is typically used in a controlled laboratory environment. It is sensitive to environmental factors such as temperature and moisture . The reagent is stored at a temperature of 2-8°C to maintain its stability . Its use is discouraged because coupling using BOP liberates HMPA which is carcinogenic .
Análisis Bioquímico
Biochemical Properties
Tri(dimethylamino)benzotriazol-1-yloxyphosphonium hexafluorophosphate plays a significant role in biochemical reactions, particularly in peptide coupling . It forms reactive intermediates which allow for the amines to bond together with little energy loss . This reagent is advantageous in peptide coupling because there are no side reactions from the dehydration of asparagine or glutamine .
Cellular Effects
The cellular effects of Tri(dimethylamino)benzotriazol-1-yloxyphosphonium hexafluorophosphate are primarily related to its role in peptide synthesis. Its use is discouraged because coupling using this reagent liberates Hexamethylphosphoramide (HMPA) which is carcinogenic .
Molecular Mechanism
The molecular mechanism of Tri(dimethylamino)benzotriazol-1-yloxyphosphonium hexafluorophosphate involves the formation of reactive intermediates in peptide coupling . These intermediates facilitate the bonding of amines with little energy loss .
Temporal Effects in Laboratory Settings
It is known that in the reduction of carboxylic acids, using this reagent with Sodium borohydride (NaBH4) resulted in high percent yields .
Metabolic Pathways
It is known that this reagent is used in the synthesis of peptides .
Métodos De Preparación
Synthetic Routes and Reaction Conditions
Benzotriazol-1-yloxytris(dimethylamino)phosphonium hexafluorophosphate can be synthesized from 1-hydroxybenzotriazole and a chlorophosphonium reagent under basic conditions . The reaction typically involves the use of a base such as triethylamine to facilitate the formation of the desired product.
Industrial Production Methods
In industrial settings, the production of benzotriazol-1-yloxytris(dimethylamino)phosphonium hexafluorophosphate follows similar synthetic routes but on a larger scale. The process is optimized for high yield and purity, often involving advanced purification techniques to ensure the compound meets the required specifications for various applications .
Análisis De Reacciones Químicas
Types of Reactions
Benzotriazol-1-yloxytris(dimethylamino)phosphonium hexafluorophosphate primarily undergoes coupling reactions, where it facilitates the formation of amide bonds. It can also be used in esterification reactions to form esters from carboxylic acids and alcohols .
Common Reagents and Conditions
Common reagents used in reactions with benzotriazol-1-yloxytris(dimethylamino)phosphonium hexafluorophosphate include carboxylic acids, amines, and alcohols. The reactions are typically carried out under mild conditions, often at room temperature, to prevent side reactions and ensure high yields .
Major Products
The major products formed from reactions involving benzotriazol-1-yloxytris(dimethylamino)phosphonium hexafluorophosphate are amides and esters. These products are valuable intermediates in the synthesis of peptides and other complex organic molecules .
Comparación Con Compuestos Similares
Similar Compounds
PyBOP: PyBOP is another phosphonium-based coupling reagent used in peptide synthesis.
HATU: HATU is a uronium-based coupling reagent that is also used in peptide synthesis.
TBTU: TBTU is another uronium-based reagent used for peptide coupling.
Uniqueness
Benzotriazol-1-yloxytris(dimethylamino)phosphonium hexafluorophosphate is unique due to its high efficiency in peptide coupling reactions and its ability to minimize side reactions such as racemization. Its versatility in various organic synthesis reactions makes it a valuable reagent in both research and industrial applications .
Propiedades
IUPAC Name |
benzotriazol-1-yloxy-tris(dimethylamino)phosphanium;hexafluorophosphate | |
---|---|---|
Source | PubChem | |
URL | https://pubchem.ncbi.nlm.nih.gov | |
Description | Data deposited in or computed by PubChem | |
InChI |
InChI=1S/C12H22N6OP.F6P/c1-15(2)20(16(3)4,17(5)6)19-18-12-10-8-7-9-11(12)13-14-18;1-7(2,3,4,5)6/h7-10H,1-6H3;/q+1;-1 | |
Source | PubChem | |
URL | https://pubchem.ncbi.nlm.nih.gov | |
Description | Data deposited in or computed by PubChem | |
InChI Key |
MGEVGECQZUIPSV-UHFFFAOYSA-N | |
Source | PubChem | |
URL | https://pubchem.ncbi.nlm.nih.gov | |
Description | Data deposited in or computed by PubChem | |
Canonical SMILES |
CN(C)[P+](N(C)C)(N(C)C)ON1C2=CC=CC=C2N=N1.F[P-](F)(F)(F)(F)F | |
Source | PubChem | |
URL | https://pubchem.ncbi.nlm.nih.gov | |
Description | Data deposited in or computed by PubChem | |
Molecular Formula |
C12H22F6N6OP2 | |
Source | PubChem | |
URL | https://pubchem.ncbi.nlm.nih.gov | |
Description | Data deposited in or computed by PubChem | |
Related CAS |
56602-32-5 (Parent) | |
Record name | Tri(dimethylamino)benzotriazol-1-yloxyphosphonium hexafluorophosphate | |
Source | ChemIDplus | |
URL | https://pubchem.ncbi.nlm.nih.gov/substance/?source=chemidplus&sourceid=0056602336 | |
Description | ChemIDplus is a free, web search system that provides access to the structure and nomenclature authority files used for the identification of chemical substances cited in National Library of Medicine (NLM) databases, including the TOXNET system. | |
DSSTOX Substance ID |
DTXSID60205170 | |
Record name | Tri(dimethylamino)benzotriazol-1-yloxyphosphonium hexafluorophosphate | |
Source | EPA DSSTox | |
URL | https://comptox.epa.gov/dashboard/DTXSID60205170 | |
Description | DSSTox provides a high quality public chemistry resource for supporting improved predictive toxicology. | |
Molecular Weight |
442.28 g/mol | |
Source | PubChem | |
URL | https://pubchem.ncbi.nlm.nih.gov | |
Description | Data deposited in or computed by PubChem | |
CAS No. |
56602-33-6 | |
Record name | BOP reagent | |
Source | CAS Common Chemistry | |
URL | https://commonchemistry.cas.org/detail?cas_rn=56602-33-6 | |
Description | CAS Common Chemistry is an open community resource for accessing chemical information. Nearly 500,000 chemical substances from CAS REGISTRY cover areas of community interest, including common and frequently regulated chemicals, and those relevant to high school and undergraduate chemistry classes. This chemical information, curated by our expert scientists, is provided in alignment with our mission as a division of the American Chemical Society. | |
Explanation | The data from CAS Common Chemistry is provided under a CC-BY-NC 4.0 license, unless otherwise stated. | |
Record name | Tri(dimethylamino)benzotriazol-1-yloxyphosphonium hexafluorophosphate | |
Source | ChemIDplus | |
URL | https://pubchem.ncbi.nlm.nih.gov/substance/?source=chemidplus&sourceid=0056602336 | |
Description | ChemIDplus is a free, web search system that provides access to the structure and nomenclature authority files used for the identification of chemical substances cited in National Library of Medicine (NLM) databases, including the TOXNET system. | |
Record name | Tri(dimethylamino)benzotriazol-1-yloxyphosphonium hexafluorophosphate | |
Source | EPA DSSTox | |
URL | https://comptox.epa.gov/dashboard/DTXSID60205170 | |
Description | DSSTox provides a high quality public chemistry resource for supporting improved predictive toxicology. | |
Record name | Tri(dimethylamino)benzotriazol-1-yloxyphosphonium hexafluorophosphate | |
Source | European Chemicals Agency (ECHA) | |
URL | https://echa.europa.eu/substance-information/-/substanceinfo/100.054.782 | |
Description | The European Chemicals Agency (ECHA) is an agency of the European Union which is the driving force among regulatory authorities in implementing the EU's groundbreaking chemicals legislation for the benefit of human health and the environment as well as for innovation and competitiveness. | |
Explanation | Use of the information, documents and data from the ECHA website is subject to the terms and conditions of this Legal Notice, and subject to other binding limitations provided for under applicable law, the information, documents and data made available on the ECHA website may be reproduced, distributed and/or used, totally or in part, for non-commercial purposes provided that ECHA is acknowledged as the source: "Source: European Chemicals Agency, http://echa.europa.eu/". Such acknowledgement must be included in each copy of the material. ECHA permits and encourages organisations and individuals to create links to the ECHA website under the following cumulative conditions: Links can only be made to webpages that provide a link to the Legal Notice page. | |
Record name | BOP reagent | |
Source | FDA Global Substance Registration System (GSRS) | |
URL | https://gsrs.ncats.nih.gov/ginas/app/beta/substances/LBD3UE345W | |
Description | The FDA Global Substance Registration System (GSRS) enables the efficient and accurate exchange of information on what substances are in regulated products. Instead of relying on names, which vary across regulatory domains, countries, and regions, the GSRS knowledge base makes it possible for substances to be defined by standardized, scientific descriptions. | |
Explanation | Unless otherwise noted, the contents of the FDA website (www.fda.gov), both text and graphics, are not copyrighted. They are in the public domain and may be republished, reprinted and otherwise used freely by anyone without the need to obtain permission from FDA. Credit to the U.S. Food and Drug Administration as the source is appreciated but not required. | |
Retrosynthesis Analysis
AI-Powered Synthesis Planning: Our tool employs the Template_relevance Pistachio, Template_relevance Bkms_metabolic, Template_relevance Pistachio_ringbreaker, Template_relevance Reaxys, Template_relevance Reaxys_biocatalysis model, leveraging a vast database of chemical reactions to predict feasible synthetic routes.
One-Step Synthesis Focus: Specifically designed for one-step synthesis, it provides concise and direct routes for your target compounds, streamlining the synthesis process.
Accurate Predictions: Utilizing the extensive PISTACHIO, BKMS_METABOLIC, PISTACHIO_RINGBREAKER, REAXYS, REAXYS_BIOCATALYSIS database, our tool offers high-accuracy predictions, reflecting the latest in chemical research and data.
Strategy Settings
Precursor scoring | Relevance Heuristic |
---|---|
Min. plausibility | 0.01 |
Model | Template_relevance |
Template Set | Pistachio/Bkms_metabolic/Pistachio_ringbreaker/Reaxys/Reaxys_biocatalysis |
Top-N result to add to graph | 6 |
Feasible Synthetic Routes
Q1: What is the primary application of Bop reagent in chemical synthesis?
A1: Bop reagent has found widespread use as a peptide coupling agent, known for its ability to facilitate the formation of amide bonds between amino acids with high yield and minimal racemization. [, , , , , ]
Q2: How does Bop reagent mediate peptide bond formation?
A2: Bop reagent acts by activating the carboxyl group of an amino acid, forming a reactive intermediate. This intermediate readily reacts with the amine group of another amino acid, leading to the formation of a peptide bond. The benzotriazole moiety in Bop reagent plays a crucial role in minimizing racemization during this process. [, , , , ]
Q3: Are there examples of challenging peptide couplings where Bop reagent excels?
A3: Yes. Bop reagent has proven particularly valuable in cases where conventional coupling agents like DCC (dicyclohexylcarbodiimide) have shown limited efficacy. For instance, it has demonstrated superior performance in the incorporation of pyroglutamic acid and Boc-His(Tos) into peptide chains, as well as in solid-phase synthesis involving sterically hindered amino acids. [, ]
Q4: Beyond peptide synthesis, what other applications have been explored for Bop reagent?
A4: Bop reagent has demonstrated versatility in mediating a variety of other chemical transformations, including:
- Lactamization Reactions: It has been successfully employed to promote the formation of lactams, particularly β-lactams, which are crucial structural motifs in many biologically active compounds. [, , ]
- Amidation Reactions: Bop reagent has been used to facilitate the formation of amides from carboxylic acids and amines, a fundamental transformation in organic synthesis. []
- Esterification Reactions: Research has shown its potential in selective esterification reactions, particularly in the context of carbohydrate chemistry. [, ]
- Nucleotidic Coupling: Bop reagent has been explored for its utility in coupling reactions involving nucleotides, although this area remains less explored. []
- C–C Bond Formation: In the presence of a palladium catalyst, BOP has been used to activate the C–OH bond of tautomerizable quinolinones, enabling C–C bond formation with boronic acids. [, ]
Q5: What is the molecular formula and weight of Bop reagent?
A5: The molecular formula of Bop reagent is C12H22F6N6OP2, and its molecular weight is 442.29 g/mol. []
Q6: What spectroscopic data are available to characterize Bop reagent?
A6: Several spectroscopic techniques can be employed to characterize Bop reagent:* 1H NMR (acetone-d6): δ 3.0 (d, 18H, JH–P = 10 Hz, NMe2), 7.9 (m, 4H, arom.)* 31P NMR (CH2Cl2): + 43.7 (s, P+), -144.2 (septet, PF6−)* IR (KBr): 1010 (P–N), 840, 770, 560 (PF6−) []
Q7: What are the solubility properties of Bop reagent?
A7: Bop reagent is insoluble in water but readily dissolves in a range of organic solvents, including: * Tetrahydrofuran (THF)* Dichloromethane (CH2Cl2)* Acetonitrile (MeCN)* Acetone* Dimethylformamide (DMF)* N-Methylpyrrolidone (NMP)* Dimethyl sulfoxide (DMSO) []
Q8: Is Bop reagent stable under standard storage conditions?
A8: Bop reagent is known to be light-sensitive. Therefore, it's essential to store it in a cool, dark, and dry place in a tightly sealed container to maintain its stability. []
Q9: Are there any safety concerns associated with the use of Bop reagent?
A9: Yes, Bop reagent should be handled with caution. It's classified as an irritant and harmful substance. []
Q10: How has Bop reagent been utilized in solid-phase peptide synthesis (SPPS)?
A10: Bop reagent has proven highly effective for peptide bond formation in SPPS, offering rapid coupling rates and high yields. It's compatible with various solid supports like Merrifield resin and Wang resin. [, , , ]
Q11: Can Bop reagent be used with unprotected amino acid side chains in SPPS?
A11: Yes, research has demonstrated the successful application of Bop reagent in SPPS with minimally protected amino acids, simplifying synthesis and reducing the need for harsh deprotection steps. For instance, it has facilitated couplings involving unprotected Boc(hydroxy)-amino acids like Boc-Thr-OH and Boc-Tyr-OH. []
Q12: Are there specific amino acids where side chain protection remains necessary even with Bop reagent?
A12: While Bop reagent allows for minimal protection strategies, some amino acids, like asparagine, may still require side-chain protection to prevent side reactions like dehydration during coupling. []
Q13: Has Bop reagent been used in the synthesis of biologically relevant cyclic peptides?
A13: Yes, Bop reagent's ability to promote on-resin cyclization has been instrumental in synthesizing cyclic peptide analogs with various biological activities, including:
- Influenza Virus Inhibitors: Cyclic peptides mimicking the loop structure of influenza virus hemagglutinin have been synthesized using Bop reagent for on-resin cyclization. []
- Growth Hormone Analogs: It has facilitated the creation of cyclic analogs of the hypoglycemic peptide fragment hGH[6–13], offering potential therapeutic applications for metabolic disorders. []
- Nerve Growth Factor Mimics: Bop reagent enabled the synthesis of cyclic peptides modeling the β-turn of nerve growth factor loop 4, providing insights into protein structure-function relationships. []
Q14: Have studies investigated the impact of Bop reagent on the biological activity of synthesized peptides?
A14: Yes, researchers have explored how using Bop reagent during synthesis influences the bioactivity of the final peptide products:
- Immunostimulating Peptides: Bop reagent was key in the synthesis of FK-156 and FK-565, two potent immunostimulating peptides. The study highlighted its effectiveness in maintaining the chiral integrity of the products. []
- Methotrexate Conjugates: The use of Bop reagent led to the successful conjugation of Methotrexate (MTX), a chemotherapy drug, to various peptide hormone carriers. These conjugates showed promise for targeted drug delivery to tumor cells. [, ]
- Cyclic GRF Analogs: Bop reagent enabled the synthesis of cyclic GRF (Growth Hormone-Releasing Factor) analogs with preserved biological activity, demonstrating its utility in creating potentially therapeutic constrained peptides. [, ]
Descargo de responsabilidad e información sobre productos de investigación in vitro
Tenga en cuenta que todos los artículos e información de productos presentados en BenchChem están destinados únicamente con fines informativos. Los productos disponibles para la compra en BenchChem están diseñados específicamente para estudios in vitro, que se realizan fuera de organismos vivos. Los estudios in vitro, derivados del término latino "in vidrio", involucran experimentos realizados en entornos de laboratorio controlados utilizando células o tejidos. Es importante tener en cuenta que estos productos no se clasifican como medicamentos y no han recibido la aprobación de la FDA para la prevención, tratamiento o cura de ninguna condición médica, dolencia o enfermedad. Debemos enfatizar que cualquier forma de introducción corporal de estos productos en humanos o animales está estrictamente prohibida por ley. Es esencial adherirse a estas pautas para garantizar el cumplimiento de los estándares legales y éticos en la investigación y experimentación.