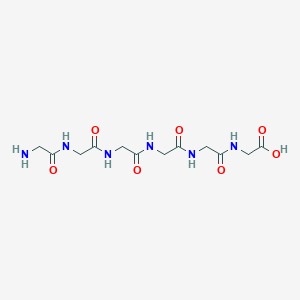
Hexaglycine
Descripción general
Descripción
La glicina es el aminoácido más simple, y su secuencia repetitiva en Gli6 da como resultado una molécula con la fórmula C12H20N6O7 y un peso molecular de 360.32 g/mol . Gli6 se utiliza principalmente en entornos de investigación y es conocido por su papel en varios estudios bioquímicos y biofísicos.
Aplicaciones Científicas De Investigación
Gli6 tiene una amplia gama de aplicaciones en la investigación científica:
Bioquímica: Utilizado como un péptido modelo para estudiar el plegamiento de proteínas, la estabilidad y las interacciones.
Biofísica: Empleo en experimentos para comprender la dinámica de los péptidos y los cambios conformacionales.
Medicina: Investigado por su posible papel en sistemas de administración de fármacos y como bloque de construcción para péptidos terapéuticos.
Industria: Utilizado en el desarrollo de materiales basados en péptidos y como sustrato en estudios enzimáticos.
Direcciones Futuras
Mecanismo De Acción
El mecanismo de acción de Gli6 está principalmente relacionado con sus propiedades estructurales como péptido. Puede interactuar con varias moléculas biológicas a través de enlaces de hidrógeno, interacciones electrostáticas y efectos hidrofóbicos. En estudios enzimáticos, Gli6 sirve como sustrato para las proteasas, que escinden los enlaces peptídicos entre los residuos de glicina. Los productos de escisión pueden luego participar en vías bioquímicas adicionales .
Compuestos Similares:
Gli2 (diglicina): Compuesto por dos residuos de glicina.
Gli3 (trigliicina): Compuesto por tres residuos de glicina.
Gli4 (tetraglicina): Compuesto por cuatro residuos de glicina.
Gli5 (pentaglicina): Compuesto por cinco residuos de glicina.
Comparación:
Longitud y Complejidad: Gli6 es más largo y más complejo que sus contrapartes más cortas, lo que puede influir en su solubilidad, estabilidad e interacciones con otras moléculas.
Actividad Biológica: La mayor longitud de Gli6 permite interacciones más extensas con los objetivos biológicos, lo que potencialmente mejora su utilidad en investigación y aplicaciones terapéuticas.
Análisis Bioquímico
Molecular Mechanism
As a peptide, it could potentially interact with biomolecules, influence enzyme activity, and cause changes in gene expression
Transport and Distribution
Peptides can be transported and distributed within cells via various mechanisms, potentially involving transporter proteins .
Subcellular Localization
Peptides can be localized to various subcellular compartments depending on their structure and function .
Métodos De Preparación
Rutas Sintéticas y Condiciones de Reacción: Gli6 se puede sintetizar utilizando técnicas estándar de síntesis de péptidos en fase sólida (SPPS). El proceso implica la adición secuencial de residuos de glicina a una cadena peptídica en crecimiento anclada a una resina sólida. La síntesis típicamente emplea la química Fmoc (9-fluorenylmetiloxicarbonil) para la protección temporal del grupo amino durante las reacciones de acoplamiento. Los pasos generales incluyen:
Desprotección: Eliminación del grupo Fmoc utilizando una base como piperidina.
Acoplamiento: Adición del siguiente residuo de glicina utilizando un reactivo de acoplamiento como HBTU (hexafluorofosfato de O-benzotriazol-N,N,N',N'-tetrametiluronio) en presencia de una base como DIPEA (N,N-diisopropiletilamina).
Escisión: Escisión final del péptido de la resina utilizando una mezcla de ácido trifluoroacético (TFA), agua y recolectores como triisopropilsilano (TIS).
Métodos de Producción Industrial: Si bien Gli6 se sintetiza principalmente para fines de investigación, la producción a escala industrial seguiría técnicas SPPS similares con optimización para lotes más grandes. Se pueden emplear sintetizadores de péptidos automatizados y de alto rendimiento para aumentar la eficiencia y el rendimiento.
Análisis De Reacciones Químicas
Tipos de Reacciones: Gli6 puede sufrir varias reacciones químicas, que incluyen:
Hidrólisis: Descomposición en residuos individuales de glicina en presencia de ácidos o bases fuertes.
Oxidación: Los residuos de glicina se pueden oxidar para formar glioxilato y amoníaco bajo condiciones específicas.
Sustitución: Los grupos amino en Gli6 pueden participar en reacciones de sustitución con electrófilos.
Reactivos y Condiciones Comunes:
Hidrólisis: Se realiza típicamente utilizando ácido clorhídrico o hidróxido de sodio a temperaturas elevadas.
Oxidación: Se puede lograr utilizando agentes oxidantes como peróxido de hidrógeno o permanganato de potasio.
Sustitución: Se pueden utilizar reactivos electrófilos como cloruros de acilo o isocianatos en condiciones suaves.
Productos Principales:
Hidrólisis: Produce residuos libres de glicina.
Oxidación: Da como resultado glioxilato y amoníaco.
Sustitución: Forma varios derivados de glicina sustituidos dependiendo del electrófilo utilizado.
Comparación Con Compuestos Similares
Gly2 (diglycine): Composed of two glycine residues.
Gly3 (triglycine): Composed of three glycine residues.
Gly4 (tetraglycine): Composed of four glycine residues.
Gly5 (pentaglycine): Composed of five glycine residues.
Comparison:
Length and Complexity: Gly6 is longer and more complex than its shorter counterparts, which can influence its solubility, stability, and interactions with other molecules.
Biological Activity: The increased length of Gly6 allows for more extensive interactions with biological targets, potentially enhancing its utility in research and therapeutic applications.
Unique Properties: Gly6’s repetitive glycine sequence provides a unique model for studying peptide behavior and interactions, making it distinct from shorter glycine peptides.
Propiedades
IUPAC Name |
2-[[2-[[2-[[2-[[2-[(2-aminoacetyl)amino]acetyl]amino]acetyl]amino]acetyl]amino]acetyl]amino]acetic acid | |
---|---|---|
Source | PubChem | |
URL | https://pubchem.ncbi.nlm.nih.gov | |
Description | Data deposited in or computed by PubChem | |
InChI |
InChI=1S/C12H20N6O7/c13-1-7(19)14-2-8(20)15-3-9(21)16-4-10(22)17-5-11(23)18-6-12(24)25/h1-6,13H2,(H,14,19)(H,15,20)(H,16,21)(H,17,22)(H,18,23)(H,24,25) | |
Source | PubChem | |
URL | https://pubchem.ncbi.nlm.nih.gov | |
Description | Data deposited in or computed by PubChem | |
InChI Key |
XJFPXLWGZWAWRQ-UHFFFAOYSA-N | |
Source | PubChem | |
URL | https://pubchem.ncbi.nlm.nih.gov | |
Description | Data deposited in or computed by PubChem | |
Canonical SMILES |
C(C(=O)NCC(=O)NCC(=O)NCC(=O)NCC(=O)NCC(=O)O)N | |
Source | PubChem | |
URL | https://pubchem.ncbi.nlm.nih.gov | |
Description | Data deposited in or computed by PubChem | |
Molecular Formula |
C12H20N6O7 | |
Source | PubChem | |
URL | https://pubchem.ncbi.nlm.nih.gov | |
Description | Data deposited in or computed by PubChem | |
DSSTOX Substance ID |
DTXSID90192125 | |
Record name | Hexaglycine | |
Source | EPA DSSTox | |
URL | https://comptox.epa.gov/dashboard/DTXSID90192125 | |
Description | DSSTox provides a high quality public chemistry resource for supporting improved predictive toxicology. | |
Molecular Weight |
360.32 g/mol | |
Source | PubChem | |
URL | https://pubchem.ncbi.nlm.nih.gov | |
Description | Data deposited in or computed by PubChem | |
CAS No. |
3887-13-6 | |
Record name | Hexaglycine | |
Source | ChemIDplus | |
URL | https://pubchem.ncbi.nlm.nih.gov/substance/?source=chemidplus&sourceid=0003887136 | |
Description | ChemIDplus is a free, web search system that provides access to the structure and nomenclature authority files used for the identification of chemical substances cited in National Library of Medicine (NLM) databases, including the TOXNET system. | |
Record name | 3887-13-6 | |
Source | DTP/NCI | |
URL | https://dtp.cancer.gov/dtpstandard/servlet/dwindex?searchtype=NSC&outputformat=html&searchlist=402061 | |
Description | The NCI Development Therapeutics Program (DTP) provides services and resources to the academic and private-sector research communities worldwide to facilitate the discovery and development of new cancer therapeutic agents. | |
Explanation | Unless otherwise indicated, all text within NCI products is free of copyright and may be reused without our permission. Credit the National Cancer Institute as the source. | |
Record name | Hexaglycine | |
Source | EPA DSSTox | |
URL | https://comptox.epa.gov/dashboard/DTXSID90192125 | |
Description | DSSTox provides a high quality public chemistry resource for supporting improved predictive toxicology. | |
Record name | HEXAGLYCINE | |
Source | FDA Global Substance Registration System (GSRS) | |
URL | https://gsrs.ncats.nih.gov/ginas/app/beta/substances/53RE43SN65 | |
Description | The FDA Global Substance Registration System (GSRS) enables the efficient and accurate exchange of information on what substances are in regulated products. Instead of relying on names, which vary across regulatory domains, countries, and regions, the GSRS knowledge base makes it possible for substances to be defined by standardized, scientific descriptions. | |
Explanation | Unless otherwise noted, the contents of the FDA website (www.fda.gov), both text and graphics, are not copyrighted. They are in the public domain and may be republished, reprinted and otherwise used freely by anyone without the need to obtain permission from FDA. Credit to the U.S. Food and Drug Administration as the source is appreciated but not required. | |
Retrosynthesis Analysis
AI-Powered Synthesis Planning: Our tool employs the Template_relevance Pistachio, Template_relevance Bkms_metabolic, Template_relevance Pistachio_ringbreaker, Template_relevance Reaxys, Template_relevance Reaxys_biocatalysis model, leveraging a vast database of chemical reactions to predict feasible synthetic routes.
One-Step Synthesis Focus: Specifically designed for one-step synthesis, it provides concise and direct routes for your target compounds, streamlining the synthesis process.
Accurate Predictions: Utilizing the extensive PISTACHIO, BKMS_METABOLIC, PISTACHIO_RINGBREAKER, REAXYS, REAXYS_BIOCATALYSIS database, our tool offers high-accuracy predictions, reflecting the latest in chemical research and data.
Strategy Settings
Precursor scoring | Relevance Heuristic |
---|---|
Min. plausibility | 0.01 |
Model | Template_relevance |
Template Set | Pistachio/Bkms_metabolic/Pistachio_ringbreaker/Reaxys/Reaxys_biocatalysis |
Top-N result to add to graph | 6 |
Feasible Synthetic Routes
Q1: What is the molecular formula and weight of Hexaglycine?
A1: this compound, with six glycine residues (C2H5NO2) linked together, possesses a molecular formula of C12H20N6O7 and a molecular weight of 360.31 g/mol.
Q2: Is there spectroscopic data available for this compound?
A2: Yes, studies utilizing techniques like circular dichroism (CD) spectroscopy have provided insights into the conformational behavior of this compound and its analogs. For instance, while exhibiting a random coil conformation in water, an analog of ACTH-(1-24)-tetracosapeptide containing this compound displayed alpha-helical characteristics in trifluoroethanol, highlighting the impact of solvent environment on its structure [].
Q3: How does the flexibility of this compound influence its end-to-end distance distribution?
A3: Research employing frequency-domain fluorescence spectroscopy has revealed that the flexibility of the peptide chain significantly impacts the end-to-end distance distribution in this compound. Specifically, the flexible nature of glycine results in a higher degree of conformational heterogeneity compared to more rigid peptides like Hexaproline. This difference in flexibility translates to a wider distribution of distances between the donor and acceptor molecules attached at the ends of the this compound chain [].
Q4: How does the presence of this compound in a fusion protein affect the protein's half-life?
A4: Studies have shown that incorporating this compound as a linker in a reiterated form of rabbit serum albumin (RSA) did not significantly alter the protein's half-life compared to a single unit of RSA []. This suggests that while albumin itself can extend the half-life of smaller proteins, simply reiterating it with a this compound linker might not confer additional benefits in terms of half-life extension.
Q5: Is the this compound peptide bond stable under strong alkaline conditions during peptide synthesis?
A5: Yes, a key advantage of using the bulky ferrocenylmethyl (Fem) group to protect glycine residues during peptide synthesis is the remarkable stability of the Gly-Gly bond in the presence of strong alkaline conditions, typically encountered during methyl ester hydrolysis steps []. This stability ensures high yields throughout the synthesis process.
Q6: Can this compound be used as a substrate to study the activity of lysostaphin-like bacteriolytic agents?
A6: Yes, this compound serves as a valuable tool for investigating the mode of action of bacteriolytic agents like zoocin A, produced by Streptococcus zooepidemicus. By observing zoocin A's ability to cleave this compound in a cell-free system, researchers have gathered evidence supporting its lysostaphin-like activity, suggesting that zoocin A targets and cleaves peptidoglycan cross-links in bacterial cell walls [].
Q7: How does the length of glycine oligopeptides affect their hydrolysis by intestinal enzymes?
A7: Studies on guinea pig intestinal mucosa have shown a correlation between the length of glycine oligopeptides and their susceptibility to enzymatic hydrolysis. While the mucosa exhibited hydrolytic activity for oligopeptides up to this compound, it did not show any activity against larger homopolypeptides []. This suggests a size limitation for the peptidase enzymes involved in the hydrolysis of glycine chains.
Q8: What is the role of this compound in studying the transport of glycine peptides in the human jejunum?
A8: Research using this compound has been crucial in determining the limits of intact absorption of glycine oligopeptides in the human jejunum. Results indicate that while di- and triglycine are readily absorbed, the uptake of glycine from this compound is comparable to that from free glycine. This suggests that longer glycine peptides are primarily hydrolyzed before absorption, highlighting the importance of peptide length for efficient nutrient uptake in the gut [].
Q9: How is this compound used in computational chemistry for studying peptide aggregation?
A9: this compound serves as a model peptide for investigating the aggregation behavior of polypeptide chains using density functional theory (DFT) calculations. By simulating the interactions of this compound strands in various conformations, researchers can gain insights into the energetic contributions of hydrogen bonding and structural distortions to the stability of different aggregated forms, such as pleated and rippled beta-sheets, collagen-like structures, and polyglycine helices [].
Q10: Can genetic algorithms be used to determine the low-energy conformations of this compound?
A10: Yes, genetic algorithms (GA) have been successfully employed to explore the conformational space of cyclic this compound and identify its low-energy conformations []. This approach involves utilizing GA parameters such as population size, number of generations, and interaction rates to effectively search for energetically favorable structures within a computationally feasible timeframe.
Descargo de responsabilidad e información sobre productos de investigación in vitro
Tenga en cuenta que todos los artículos e información de productos presentados en BenchChem están destinados únicamente con fines informativos. Los productos disponibles para la compra en BenchChem están diseñados específicamente para estudios in vitro, que se realizan fuera de organismos vivos. Los estudios in vitro, derivados del término latino "in vidrio", involucran experimentos realizados en entornos de laboratorio controlados utilizando células o tejidos. Es importante tener en cuenta que estos productos no se clasifican como medicamentos y no han recibido la aprobación de la FDA para la prevención, tratamiento o cura de ninguna condición médica, dolencia o enfermedad. Debemos enfatizar que cualquier forma de introducción corporal de estos productos en humanos o animales está estrictamente prohibida por ley. Es esencial adherirse a estas pautas para garantizar el cumplimiento de los estándares legales y éticos en la investigación y experimentación.