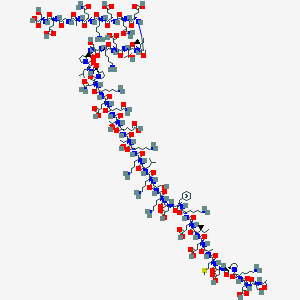
Timosina beta 4
Descripción general
Descripción
Thymosin beta4 is a peptide consisting of 43 amino acids and is encoded by the TMSB4X gene in humans . It is a major cellular constituent in many tissues and plays a crucial role in the regulation of actin polymerization . Thymosin beta4 is involved in various biological processes, including cell proliferation, migration, and differentiation . It is widely distributed in tissues such as the thymus, spleen, and peritoneal macrophages .
Aplicaciones Científicas De Investigación
Thymosin beta4 has a wide range of scientific research applications:
Chemistry: Used as a model peptide in studies of peptide synthesis and purification techniques.
Biology: Plays a role in cell migration, proliferation, and differentiation studies.
Medicine: Investigated for its therapeutic potential in wound healing, myocardial infarction, and ischemia-reperfusion injury.
Industry: Utilized in the development of peptide-based therapeutics and cosmetic products.
Mecanismo De Acción
Thymosin Beta4 (Tβ4), also known as Timbetasin, is a multifunctional peptide that plays a pivotal role in several physiological and pathological processes in the body .
Target of Action
Tβ4’s primary target is the actin cytoskeleton . Actin is a protein that forms microfilaments, which are key components of the cell’s structure and play a crucial role in cell movement, division, and shape . Tβ4 is an actin sequestering protein, which means it binds to actin monomers and prevents them from polymerizing into actin filaments .
Mode of Action
Tβ4 interacts with its target, actin, by binding to actin monomers in a 1:1 ratio . This interaction inhibits the polymerization of actin, thereby regulating the balance of depolymerization and polymerization of actin filaments . This process is essential for various cellular activities, especially cell motility, development, and differentiation .
Biochemical Pathways
Tβ4 affects several biochemical pathways. It promotes angiogenesis, enhances endothelial progenitor cell viability, and triggers the proliferation and migration of cells . It also upregulates the expression of Vascular Endothelial Growth Factor (VEGF), an important factor that promotes angiogenesis . Additionally, Tβ4 stimulates Wnt ligands on the cytomembrane, leading to the accumulation of unphosphorylated β-catenin in the cytoplasm .
Result of Action
The interaction of Tβ4 with actin results in several molecular and cellular effects. It regulates inflammation and tumor metastasis, promotes angiogenesis, wound healing, regeneration of hair follicles, and improves bone formation and tooth growth . It also plays a role in cell proliferation, migration, and differentiation .
Action Environment
The action of Tβ4 can be influenced by various environmental factors. For instance, hypoxia-inducible nitric oxide and Hypoxia-Inducible Factor-1α (HIF-1α) have been found to regulate Tβ4 expression . .
Safety and Hazards
Direcciones Futuras
Thymosin beta4 has been effectively utilized for several indications in animal experiments or clinical trials, such as myocardial infarction and myocardial ischemia-reperfusion injury, xerophthalmia, liver and renal fibrosis, ulcerative colitis and colon cancer, and skin trauma . Recent studies have reported the potential application of Tβ4 and its underlying mechanisms . Therefore, the discovery and postnatal administration of developmentally relevant agents along with other approaches may result in reversing the aging process .
Análisis Bioquímico
Biochemical Properties
Thymosin beta4 is a 43-amino acid peptide that interacts with several enzymes, proteins, and other biomolecules. One of its primary roles is to bind to actin monomers (G-actin), preventing their polymerization into filaments (F-actin). This interaction is crucial for maintaining the cytoskeleton’s integrity and regulating cell motility . Thymosin beta4 also interacts with vascular endothelial growth factor (VEGF), enhancing angiogenesis and promoting endothelial cell proliferation . Additionally, it modulates the activity of matrix metalloproteinases (MMPs), which are involved in tissue remodeling and repair .
Cellular Effects
Thymosin beta4 exerts significant effects on various cell types and cellular processes. It promotes cell migration, proliferation, and differentiation, which are essential for tissue repair and regeneration . In endothelial cells, Thymosin beta4 enhances angiogenesis by upregulating VEGF expression . It also inhibits apoptosis and reduces inflammation in different cell types, including fibroblasts and epithelial cells . Furthermore, Thymosin beta4 influences cell signaling pathways, such as the Wnt/β-catenin pathway, which is involved in cell proliferation and differentiation .
Molecular Mechanism
At the molecular level, Thymosin beta4 exerts its effects through various mechanisms. It binds to G-actin in a 1:1 ratio, preventing its polymerization into F-actin and thus regulating the cytoskeleton’s dynamics . Thymosin beta4 also interacts with VEGF, promoting angiogenesis and endothelial cell proliferation . Additionally, it modulates the activity of MMPs, facilitating tissue remodeling and repair . Thymosin beta4’s anti-inflammatory effects are mediated through the inhibition of nuclear factor-kappa B (NF-κB) signaling, reducing the expression of pro-inflammatory cytokines .
Temporal Effects in Laboratory Settings
In laboratory settings, the effects of Thymosin beta4 have been observed to change over time. Studies have shown that Thymosin beta4 promotes cell migration and proliferation within hours of treatment . Its stability and degradation depend on the experimental conditions, with some studies reporting a half-life of several hours . Long-term effects of Thymosin beta4 include sustained angiogenesis and tissue repair, as well as reduced inflammation and fibrosis .
Dosage Effects in Animal Models
The effects of Thymosin beta4 vary with different dosages in animal models. At low doses, Thymosin beta4 promotes wound healing and tissue repair without significant adverse effects . At higher doses, it may cause toxic effects, such as increased inflammation and tissue damage . Studies have shown that the optimal dosage for therapeutic effects ranges from 1 to 10 mg/kg, depending on the specific application and animal model .
Metabolic Pathways
Thymosin beta4 is involved in several metabolic pathways, including actin polymerization and angiogenesis . It interacts with enzymes such as MMPs and VEGF, modulating their activity and promoting tissue repair . Thymosin beta4 also influences metabolic flux by regulating the expression of genes involved in cell proliferation and differentiation . Additionally, it affects metabolite levels, such as reducing the production of pro-inflammatory cytokines .
Transport and Distribution
Thymosin beta4 is transported and distributed within cells and tissues through various mechanisms. It binds to actin monomers in the cytoplasm, preventing their polymerization and regulating the cytoskeleton’s dynamics . Thymosin beta4 is also transported to the nucleus, where it may influence gene expression and cell signaling pathways . Additionally, it interacts with transporters and binding proteins that facilitate its distribution within different tissues .
Subcellular Localization
Thymosin beta4 is localized in various subcellular compartments, including the cytoplasm and nucleus . In the cytoplasm, it binds to G-actin, preventing its polymerization into F-actin and regulating the cytoskeleton’s dynamics . Thymosin beta4 is also found in the nucleus, where it may influence gene expression and cell signaling pathways . Its subcellular localization is regulated by targeting signals and post-translational modifications that direct it to specific compartments or organelles .
Métodos De Preparación
Synthetic Routes and Reaction Conditions: Thymosin beta4 can be synthesized using solid-phase peptide synthesis (SPPS), a method that allows the sequential addition of amino acids to a growing peptide chain anchored to a solid resin . The synthesis involves the use of protected amino acids, coupling reagents, and deprotection steps to ensure the correct sequence and structure of the peptide .
Industrial Production Methods: Industrial production of thymosin beta4 involves large-scale SPPS, followed by purification using high-performance liquid chromatography (HPLC) to achieve high purity . The peptide is then lyophilized to obtain a stable powder form suitable for various applications .
Análisis De Reacciones Químicas
Types of Reactions: Thymosin beta4 primarily undergoes peptide bond formation and cleavage reactions during its synthesis and degradation . It does not typically participate in oxidation, reduction, or substitution reactions due to its peptide nature .
Common Reagents and Conditions:
Coupling Reagents: N,N’-Diisopropylcarbodiimide (DIC), O-Benzotriazole-N,N,N’,N’-tetramethyl-uronium-hexafluoro-phosphate (HBTU).
Deprotection Reagents: Trifluoroacetic acid (TFA), piperidine.
Purification: High-performance liquid chromatography (HPLC).
Major Products: The primary product of thymosin beta4 synthesis is the peptide itself, with high purity achieved through purification steps .
Comparación Con Compuestos Similares
Thymosin beta10: Another member of the thymosin beta family, involved in similar biological processes.
Thymosin beta15: Shares structural similarities with thymosin beta4 and is involved in actin regulation.
Uniqueness: Thymosin beta4 is unique due to its high abundance in various tissues and its significant role in actin regulation . Its ability to promote angiogenesis and wound healing makes it a valuable peptide in therapeutic applications .
Thymosin beta4 stands out among its counterparts for its extensive distribution and multifunctional roles in the body .
Propiedades
IUPAC Name |
(4S)-4-[[2-[[(2S)-2-[[(2S)-2-[[(2S)-2-[[(2S)-2-[[(2S)-2-[[(2S)-2-[[(2S,3S)-2-[[(2S,3R)-2-[[(2S)-2-[[(2S)-2-[[(2S)-2-[[(2S)-1-[(2S)-2-[[(2S)-1-[(2S)-2-[[(2S)-2-[[(2S)-2-[[(2S)-2-[[(2S,3R)-2-[[(2S)-2-[[(2S,3R)-2-[[(2S)-2-[[(2S)-2-[[(2S)-2-[[(2S)-2-[[(2S)-2-[[(2S)-2-[[(2S)-2-[[(2S)-2-[[(2S)-2-[[(2S)-2-[[(2S,3S)-2-[[(2S)-2-[[(2S)-2-[[(2S)-2-[[(2S)-2-[[(2S)-1-[(2S)-2-[[(2S)-2-[[(2S)-2-acetamido-3-hydroxypropanoyl]amino]-3-carboxypropanoyl]amino]-6-aminohexanoyl]pyrrolidine-2-carbonyl]amino]-3-carboxypropanoyl]amino]-4-methylsulfanylbutanoyl]amino]propanoyl]amino]-4-carboxybutanoyl]amino]-3-methylpentanoyl]amino]-4-carboxybutanoyl]amino]-6-aminohexanoyl]amino]-3-phenylpropanoyl]amino]-3-carboxypropanoyl]amino]-6-aminohexanoyl]amino]-3-hydroxypropanoyl]amino]-6-aminohexanoyl]amino]-4-methylpentanoyl]amino]-6-aminohexanoyl]amino]-6-aminohexanoyl]amino]-3-hydroxybutanoyl]amino]-4-carboxybutanoyl]amino]-3-hydroxybutanoyl]amino]-5-amino-5-oxopentanoyl]amino]-4-carboxybutanoyl]amino]-6-aminohexanoyl]amino]-4-amino-4-oxobutanoyl]pyrrolidine-2-carbonyl]amino]-4-methylpentanoyl]pyrrolidine-2-carbonyl]amino]-3-hydroxypropanoyl]amino]-6-aminohexanoyl]amino]-4-carboxybutanoyl]amino]-3-hydroxybutanoyl]amino]-3-methylpentanoyl]amino]-4-carboxybutanoyl]amino]-5-amino-5-oxopentanoyl]amino]-4-carboxybutanoyl]amino]-6-aminohexanoyl]amino]-5-amino-5-oxopentanoyl]amino]propanoyl]amino]acetyl]amino]-5-[[(1S)-1-carboxy-2-hydroxyethyl]amino]-5-oxopentanoic acid | |
---|---|---|
Source | PubChem | |
URL | https://pubchem.ncbi.nlm.nih.gov | |
Description | Data deposited in or computed by PubChem | |
InChI |
InChI=1S/C212H350N56O78S/c1-16-106(7)166(261-190(323)131(64-75-160(292)293)231-172(305)109(10)228-174(307)134(78-91-347-15)245-196(329)140(98-165(302)303)255-201(334)147-53-39-88-266(147)209(342)135(52-29-38-87-221)250-197(330)139(97-164(300)301)254-198(331)143(100-269)229-113(14)276)204(337)246-129(62-73-158(288)289)187(320)233-118(47-24-33-82-216)179(312)252-137(94-114-42-19-18-20-43-114)194(327)253-138(96-163(298)299)195(328)237-121(50-27-36-85-219)181(314)258-144(101-270)199(332)239-119(48-25-34-83-217)178(311)251-136(92-104(3)4)193(326)236-116(45-22-31-80-214)175(308)235-122(51-28-37-86-220)189(322)263-168(110(11)273)207(340)249-133(66-77-162(296)297)192(325)264-169(111(12)274)206(339)248-126(58-69-152(224)279)184(317)243-128(61-72-157(286)287)186(319)234-120(49-26-35-84-218)180(313)256-142(95-153(225)280)211(344)268-90-40-54-148(268)202(335)257-141(93-105(5)6)210(343)267-89-41-55-149(267)203(336)259-145(102-271)200(333)238-117(46-23-32-81-215)177(310)244-132(65-76-161(294)295)191(324)265-170(112(13)275)208(341)262-167(107(8)17-2)205(338)247-130(63-74-159(290)291)188(321)241-125(57-68-151(223)278)183(316)242-127(60-71-156(284)285)185(318)232-115(44-21-30-79-213)176(309)240-124(56-67-150(222)277)173(306)227-108(9)171(304)226-99-154(281)230-123(59-70-155(282)283)182(315)260-146(103-272)212(345)346/h18-20,42-43,104-112,115-149,166-170,269-275H,16-17,21-41,44-103,213-221H2,1-15H3,(H2,222,277)(H2,223,278)(H2,224,279)(H2,225,280)(H,226,304)(H,227,306)(H,228,307)(H,229,276)(H,230,281)(H,231,305)(H,232,318)(H,233,320)(H,234,319)(H,235,308)(H,236,326)(H,237,328)(H,238,333)(H,239,332)(H,240,309)(H,241,321)(H,242,316)(H,243,317)(H,244,310)(H,245,329)(H,246,337)(H,247,338)(H,248,339)(H,249,340)(H,250,330)(H,251,311)(H,252,312)(H,253,327)(H,254,331)(H,255,334)(H,256,313)(H,257,335)(H,258,314)(H,259,336)(H,260,315)(H,261,323)(H,262,341)(H,263,322)(H,264,325)(H,265,324)(H,282,283)(H,284,285)(H,286,287)(H,288,289)(H,290,291)(H,292,293)(H,294,295)(H,296,297)(H,298,299)(H,300,301)(H,302,303)(H,345,346)/t106-,107-,108-,109-,110+,111+,112+,115-,116-,117-,118-,119-,120-,121-,122-,123-,124-,125-,126-,127-,128-,129-,130-,131-,132-,133-,134-,135-,136-,137-,138-,139-,140-,141-,142-,143-,144-,145-,146-,147-,148-,149-,166-,167-,168-,169-,170-/m0/s1 | |
Source | PubChem | |
URL | https://pubchem.ncbi.nlm.nih.gov | |
Description | Data deposited in or computed by PubChem | |
InChI Key |
UGPMCIBIHRSCBV-XNBOLLIBSA-N | |
Source | PubChem | |
URL | https://pubchem.ncbi.nlm.nih.gov | |
Description | Data deposited in or computed by PubChem | |
Canonical SMILES |
CCC(C)C(C(=O)NC(CCC(=O)O)C(=O)NC(CCCCN)C(=O)NC(CC1=CC=CC=C1)C(=O)NC(CC(=O)O)C(=O)NC(CCCCN)C(=O)NC(CO)C(=O)NC(CCCCN)C(=O)NC(CC(C)C)C(=O)NC(CCCCN)C(=O)NC(CCCCN)C(=O)NC(C(C)O)C(=O)NC(CCC(=O)O)C(=O)NC(C(C)O)C(=O)NC(CCC(=O)N)C(=O)NC(CCC(=O)O)C(=O)NC(CCCCN)C(=O)NC(CC(=O)N)C(=O)N2CCCC2C(=O)NC(CC(C)C)C(=O)N3CCCC3C(=O)NC(CO)C(=O)NC(CCCCN)C(=O)NC(CCC(=O)O)C(=O)NC(C(C)O)C(=O)NC(C(C)CC)C(=O)NC(CCC(=O)O)C(=O)NC(CCC(=O)N)C(=O)NC(CCC(=O)O)C(=O)NC(CCCCN)C(=O)NC(CCC(=O)N)C(=O)NC(C)C(=O)NCC(=O)NC(CCC(=O)O)C(=O)NC(CO)C(=O)O)NC(=O)C(CCC(=O)O)NC(=O)C(C)NC(=O)C(CCSC)NC(=O)C(CC(=O)O)NC(=O)C4CCCN4C(=O)C(CCCCN)NC(=O)C(CC(=O)O)NC(=O)C(CO)NC(=O)C | |
Source | PubChem | |
URL | https://pubchem.ncbi.nlm.nih.gov | |
Description | Data deposited in or computed by PubChem | |
Isomeric SMILES |
CC[C@H](C)[C@@H](C(=O)N[C@@H](CCC(=O)O)C(=O)N[C@@H](CCCCN)C(=O)N[C@@H](CC1=CC=CC=C1)C(=O)N[C@@H](CC(=O)O)C(=O)N[C@@H](CCCCN)C(=O)N[C@@H](CO)C(=O)N[C@@H](CCCCN)C(=O)N[C@@H](CC(C)C)C(=O)N[C@@H](CCCCN)C(=O)N[C@@H](CCCCN)C(=O)N[C@@H]([C@@H](C)O)C(=O)N[C@@H](CCC(=O)O)C(=O)N[C@@H]([C@@H](C)O)C(=O)N[C@@H](CCC(=O)N)C(=O)N[C@@H](CCC(=O)O)C(=O)N[C@@H](CCCCN)C(=O)N[C@@H](CC(=O)N)C(=O)N2CCC[C@H]2C(=O)N[C@@H](CC(C)C)C(=O)N3CCC[C@H]3C(=O)N[C@@H](CO)C(=O)N[C@@H](CCCCN)C(=O)N[C@@H](CCC(=O)O)C(=O)N[C@@H]([C@@H](C)O)C(=O)N[C@@H]([C@@H](C)CC)C(=O)N[C@@H](CCC(=O)O)C(=O)N[C@@H](CCC(=O)N)C(=O)N[C@@H](CCC(=O)O)C(=O)N[C@@H](CCCCN)C(=O)N[C@@H](CCC(=O)N)C(=O)N[C@@H](C)C(=O)NCC(=O)N[C@@H](CCC(=O)O)C(=O)N[C@@H](CO)C(=O)O)NC(=O)[C@H](CCC(=O)O)NC(=O)[C@H](C)NC(=O)[C@H](CCSC)NC(=O)[C@H](CC(=O)O)NC(=O)[C@@H]4CCCN4C(=O)[C@H](CCCCN)NC(=O)[C@H](CC(=O)O)NC(=O)[C@H](CO)NC(=O)C | |
Source | PubChem | |
URL | https://pubchem.ncbi.nlm.nih.gov | |
Description | Data deposited in or computed by PubChem | |
Molecular Formula |
C212H350N56O78S | |
Source | PubChem | |
URL | https://pubchem.ncbi.nlm.nih.gov | |
Description | Data deposited in or computed by PubChem | |
DSSTOX Substance ID |
DTXSID70228259 | |
Record name | Thymosin beta(4) | |
Source | EPA DSSTox | |
URL | https://comptox.epa.gov/dashboard/DTXSID70228259 | |
Description | DSSTox provides a high quality public chemistry resource for supporting improved predictive toxicology. | |
Molecular Weight |
4963 g/mol | |
Source | PubChem | |
URL | https://pubchem.ncbi.nlm.nih.gov | |
Description | Data deposited in or computed by PubChem | |
CAS No. |
77591-33-4 | |
Record name | Timbetasin [USAN] | |
Source | ChemIDplus | |
URL | https://pubchem.ncbi.nlm.nih.gov/substance/?source=chemidplus&sourceid=0077591334 | |
Description | ChemIDplus is a free, web search system that provides access to the structure and nomenclature authority files used for the identification of chemical substances cited in National Library of Medicine (NLM) databases, including the TOXNET system. | |
Record name | Thymosin beta(4) | |
Source | EPA DSSTox | |
URL | https://comptox.epa.gov/dashboard/DTXSID70228259 | |
Description | DSSTox provides a high quality public chemistry resource for supporting improved predictive toxicology. | |
Descargo de responsabilidad e información sobre productos de investigación in vitro
Tenga en cuenta que todos los artículos e información de productos presentados en BenchChem están destinados únicamente con fines informativos. Los productos disponibles para la compra en BenchChem están diseñados específicamente para estudios in vitro, que se realizan fuera de organismos vivos. Los estudios in vitro, derivados del término latino "in vidrio", involucran experimentos realizados en entornos de laboratorio controlados utilizando células o tejidos. Es importante tener en cuenta que estos productos no se clasifican como medicamentos y no han recibido la aprobación de la FDA para la prevención, tratamiento o cura de ninguna condición médica, dolencia o enfermedad. Debemos enfatizar que cualquier forma de introducción corporal de estos productos en humanos o animales está estrictamente prohibida por ley. Es esencial adherirse a estas pautas para garantizar el cumplimiento de los estándares legales y éticos en la investigación y experimentación.