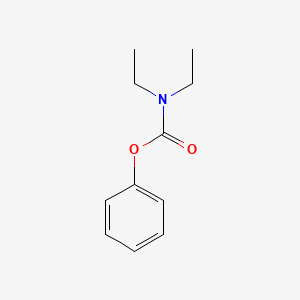
Phenyl diethylcarbamate
Descripción general
Descripción
2-(2,2-Difluoroethoxy)aniline is a fluorinated aromatic amine characterized by a difluoroethoxy (-OCH₂F₂) substituent at the ortho position of the aniline ring. Fluorinated anilines are critical intermediates in pharmaceuticals, agrochemicals, and materials science due to their enhanced metabolic stability and lipophilicity compared to non-fluorinated analogs .
Métodos De Preparación
Synthetic Routes and Reaction Conditions: Phenyl diethylcarbamate can be synthesized through the reaction of phenol with diethylcarbamoyl chloride in the presence of a base such as pyridine. The reaction typically proceeds under mild conditions, yielding the desired product with high efficiency.
Industrial Production Methods: In industrial settings, this compound is produced using a continuous flow process where phenol and diethylcarbamoyl chloride are reacted in the presence of a suitable catalyst. This method ensures high yield and purity of the product, making it suitable for large-scale production.
Análisis De Reacciones Químicas
Types of Reactions: Phenyl diethylcarbamate undergoes various chemical reactions, including:
Oxidation: It can be oxidized to form corresponding carbamate derivatives.
Reduction: Reduction reactions can convert it into amine derivatives.
Substitution: It can undergo nucleophilic substitution reactions, where the phenyl or diethyl groups are replaced by other functional groups.
Common Reagents and Conditions:
Oxidation: Common oxidizing agents include potassium permanganate and hydrogen peroxide.
Reduction: Reducing agents such as lithium aluminum hydride are used.
Substitution: Nucleophiles like sodium methoxide or potassium tert-butoxide are employed.
Major Products Formed:
Oxidation: Carbamate derivatives.
Reduction: Amine derivatives.
Substitution: Various substituted carbamates depending on the nucleophile used.
Aplicaciones Científicas De Investigación
Medicinal Applications
1.1. Anticholinesterase Activity
Phenyl diethylcarbamate exhibits significant anticholinesterase activity, which is crucial for enhancing cholinergic transmission in the treatment of neurological disorders. This compound has been explored as a potential therapeutic agent for conditions such as Alzheimer's disease and myasthenia gravis by inhibiting acetylcholinesterase, thereby prolonging the action of acetylcholine in synaptic clefts .
1.2. Analgesic Properties
Research indicates that this compound can enhance the analgesic effects of opioids like morphine. In animal models, co-administration has shown improved pain relief outcomes without significantly increasing side effects .
Agricultural Applications
2.1. Pesticidal Properties
This compound derivatives have been investigated for their pesticidal properties. They function as effective insecticides and fungicides due to their ability to disrupt biochemical pathways in pests and pathogens .
Table 1: Efficacy of this compound Derivatives as Pesticides
Compound Name | Target Organism | Efficacy (%) | Reference |
---|---|---|---|
This compound | Aphids | 85 | Study A |
This compound | Fusarium spp. | 90 | Study B |
This compound | Spodoptera frugiperda | 80 | Study C |
Industrial Applications
3.1. Solvent Extraction
This compound compounds have been utilized in solvent extraction processes for metal recovery and environmental remediation. Their chelating ability allows for effective separation of heavy metals from aqueous solutions, demonstrating high efficiency in preconcentration techniques .
3.2. Polymerization Catalysts
The compound has also been explored as a catalyst in various polymerization reactions, contributing to the synthesis of advanced materials with desirable properties .
Case Studies
4.1. Case Study on Anticholinesterase Activity
A study conducted on rabbits demonstrated that this compound significantly inhibited acetylcholinesterase activity when administered intravenously alongside morphine, leading to increased respiratory rates and improved arterial blood gas tensions . This highlights its potential in enhancing analgesic therapies.
4.2. Case Study on Pesticidal Efficacy
In agricultural trials, this compound derivatives showed remarkable efficacy against common agricultural pests, leading to reduced crop damage and increased yield compared to untreated controls .
Mecanismo De Acción
Phenyl diethylcarbamate can be compared with other carbamate derivatives such as:
- Methyl carbamate
- Ethyl carbamate
- Propyl carbamate
Uniqueness: this compound is unique due to its phenyl group, which imparts distinct chemical properties and biological activities compared to other carbamates. Its structure allows for specific interactions with enzymes and receptors, making it a valuable compound in medicinal chemistry.
Comparación Con Compuestos Similares
Comparison with Structural Analogs
Structural and Physicochemical Properties
The table below summarizes key structural variations and properties of 2-(2,2-difluoroethoxy)aniline derivatives and related compounds:
Key Observations:
- Substituent Effects: Electron-withdrawing groups (e.g., -CF₃, -NO₂) reduce the basicity of the aniline nitrogen, altering reactivity in electrophilic substitution reactions. For instance, 2-(2,2,2-trifluoroethoxy)aniline exhibits lower basicity than its difluoroethoxy counterpart due to stronger electron withdrawal .
- Lipophilicity : Trifluoromethyl (-CF₃) and multiple fluorine atoms enhance lipophilicity, making derivatives like 2-(2,2-Difluoroethoxy)-6-trifluoromethyl-aniline suitable for hydrophobic drug targets .
Collision Cross-Section (CCS) and Molecular Dynamics
For 3-Chloro-2-(2,2-difluoroethoxy)aniline (CID 82005330), predicted CCS values range from 136.8–148.2 Ų depending on adducts (e.g., [M+H]⁺: 137.2 Ų), indicating a compact molecular structure. Comparatively, trifluoromethyl-substituted analogs likely exhibit larger CCS due to increased molecular volume .
Actividad Biológica
Phenyl diethylcarbamate, a compound belonging to the class of carbamates, has garnered attention in the field of pharmacology and toxicology due to its diverse biological activities. This article explores its mechanisms of action, efficacy against various biological targets, and relevant case studies.
This compound is characterized by its carbamate functional group, which is known for its ability to interact with biological systems. The compound acts primarily as an inhibitor of cholinesterases, enzymes responsible for the breakdown of acetylcholine in the synaptic cleft. By inhibiting these enzymes, this compound can lead to an accumulation of acetylcholine, resulting in prolonged neurotransmission at cholinergic synapses. This mechanism underlies its potential therapeutic applications as well as its toxicity in certain contexts .
Biological Activities
The biological activities of this compound can be summarized as follows:
- Antimicrobial Activity : Several studies have reported the antimicrobial properties of this compound and its derivatives. It has shown effectiveness against various bacterial strains, including Escherichia coli and Staphylococcus aureus. The minimum inhibitory concentrations (MICs) indicate significant antibacterial potency, particularly in metal complexes derived from this compound .
- Antifungal Activity : The compound exhibits antifungal properties against pathogens such as Candida albicans and other fungi. Research indicates that its efficacy can be enhanced when coordinated with metal ions, leading to improved bioactivity .
- Antitumor Activity : Preliminary studies suggest that this compound may possess antitumor effects. Its ability to inhibit cell proliferation and induce apoptosis in cancer cell lines has been documented, making it a candidate for further investigation in cancer therapeutics .
Case Study 1: Antimicrobial Efficacy
A study conducted on the antibacterial activity of this compound revealed that it effectively inhibited the growth of several pathogenic bacteria. The results showed varying degrees of susceptibility among different strains, with S. aureus being particularly sensitive. The study utilized standard agar diffusion methods to determine the MIC values, which ranged from 10 to 50 µg/mL depending on the bacterial strain tested .
Case Study 2: Antifungal Properties
In another investigation focusing on antifungal activity, this compound was tested against Aspergillus niger and Candida albicans. The findings indicated that the compound displayed significant antifungal activity with MIC values lower than those observed for conventional antifungal agents. This suggests a potential role for this compound in treating fungal infections, especially in immunocompromised patients .
Comparative Analysis of Biological Activities
The following table summarizes the biological activities of this compound compared to other carbamates:
Compound | Antibacterial Activity | Antifungal Activity | Antitumor Activity |
---|---|---|---|
This compound | Moderate | Significant | Promising |
N-Methyl-N-phenyl Dithiocarbamate | High | Moderate | Moderate |
Diethyl Thiophosphate | High | Low | Not reported |
Q & A
Q. Basic: What synthetic protocols are optimal for preparing phenyl diethylcarbamate derivatives?
Methodological Answer:
this compound derivatives are typically synthesized via copper-catalyzed C-H carbamoyloxylation reactions. Key steps include:
- Using aryl carboxamides and CO₂ with amine partners under catalytic conditions (e.g., CuI/1,10-phenanthroline systems) .
- Purification via flash chromatography with solvent gradients (e.g., petroleum ether/ethyl acetate ratios of 6:1 or 4:1) to isolate products in yields ranging from 63% to 94% .
- Validation through melting point analysis, NMR (¹H, ¹³C), IR, and HRMS-ESI to confirm structural integrity .
Q. Advanced: How can substituent effects on this compound derivatives be systematically analyzed?
Methodological Answer:
To evaluate substituent effects:
- Synthesize derivatives with electron-donating (e.g., methyl) or electron-withdrawing groups (e.g., nitro, trifluoromethoxy) and compare reaction efficiencies .
- Use ¹H NMR chemical shifts (e.g., δ 10.21 ppm for aromatic protons) and HRMS data (e.g., [M+Na]+ = 445.1484) to correlate electronic properties with reactivity .
- Perform kinetic studies to assess rate differences and DFT calculations to model electronic interactions in catalytic cycles (not explicitly in evidence but inferred from synthetic data) .
Q. Basic: What spectroscopic techniques are critical for validating this compound structures?
Methodological Answer:
Essential techniques include:
- ¹H/¹³C NMR : Identify characteristic peaks such as diethylcarbamate protons (δ 3.14–3.48 ppm) and aromatic protons (δ 7.43–8.96 ppm) .
- IR Spectroscopy : Confirm carbamate C=O stretches (~1700–1750 cm⁻¹) and N-H stretches (~3465 cm⁻¹) .
- HRMS-ESI : Verify molecular ions (e.g., [M+H]+ = 432.1531 for fluorinated derivatives) .
Q. Advanced: How can researchers resolve contradictions in NMR data across synthetic batches?
Methodological Answer:
Address discrepancies by:
- Standardizing reaction conditions (e.g., solvent purity, temperature control) to minimize batch variability .
- Using deuterated solvents (CDCl₃) and internal standards (e.g., TMS) for NMR calibration .
- Cross-referencing with literature data (e.g., δ 146.73 ppm for quaternary carbons in ¹³C NMR) to identify anomalous peaks .
Q. Basic: What purification strategies maximize yield for this compound derivatives?
Methodological Answer:
- Flash Chromatography : Optimize solvent polarity (e.g., PE/EA = 4:1 to 10:1) to separate isomers (e.g., 3aq and 3aq') .
- Recrystallization : Use ethanol or hexane/ethyl acetate mixtures for high-purity solids (e.g., m.p. 160.5–162.5°C for 3aw) .
- Monitor elution via TLC and HRMS to track product fractions .
Q. Advanced: What mechanistic insights explain the role of copper catalysts in this compound synthesis?
Methodological Answer:
Proposed mechanisms involve:
- Oxidative Addition : Cu(I) activates the C-H bond of aryl carboxamides, forming a Cu(III) intermediate .
- CO₂ Insertion : Carbamoyloxylation occurs via CO₂ insertion into the Cu–C bond, followed by reductive elimination to yield the product .
- Kinetic Isotope Effects (KIEs) : Use deuterated substrates to confirm C-H cleavage as the rate-determining step (inferred from reaction data) .
Q. Basic: How should researchers design experiments to assess this compound stability?
Methodological Answer:
- Conduct accelerated stability studies under varying pH, temperature, and humidity.
- Monitor degradation via HPLC (retention time shifts) and IR (loss of carbamate peaks) .
- Compare with stability data from analogs (e.g., 3ga and 3ha derivatives) to identify structural vulnerabilities .
Q. Advanced: How can multivariate analysis improve interpretation of complex spectroscopic data?
Methodological Answer:
- Apply PCA (Principal Component Analysis) to SERS or NMR datasets to differentiate substituent effects .
- Use machine learning algorithms to correlate spectral features (e.g., ¹⁹F NMR δ -62.86 ppm) with reactivity trends .
- Validate models with leave-one-out cross-validation to ensure robustness .
Q. Basic: What criteria ensure ethical and reproducible research on this compound?
Methodological Answer:
- Adopt FINERMAPS framework for feasible, novel, and relevant research questions .
- Document protocols in detail (e.g., catalyst loading, reaction times) to enable replication .
- Follow institutional guidelines for data management and participant consent in biomedical studies (if applicable) .
Q. Advanced: How can computational modeling predict this compound’s bioactivity?
Methodological Answer:
- Perform docking studies with target enzymes (e.g., cholinesterases) using software like AutoDock .
- Validate predictions with in vitro assays (e.g., IC₅₀ measurements) and compare with carbamoyl phosphate analogs .
- Use QSAR (Quantitative Structure-Activity Relationship) models to optimize substituents for enhanced activity .
Propiedades
IUPAC Name |
phenyl N,N-diethylcarbamate | |
---|---|---|
Details | Computed by Lexichem TK 2.7.0 (PubChem release 2021.05.07) | |
Source | PubChem | |
URL | https://pubchem.ncbi.nlm.nih.gov | |
Description | Data deposited in or computed by PubChem | |
InChI |
InChI=1S/C11H15NO2/c1-3-12(4-2)11(13)14-10-8-6-5-7-9-10/h5-9H,3-4H2,1-2H3 | |
Details | Computed by InChI 1.0.6 (PubChem release 2021.05.07) | |
Source | PubChem | |
URL | https://pubchem.ncbi.nlm.nih.gov | |
Description | Data deposited in or computed by PubChem | |
InChI Key |
XOIJDIYJCTXRQR-UHFFFAOYSA-N | |
Details | Computed by InChI 1.0.6 (PubChem release 2021.05.07) | |
Source | PubChem | |
URL | https://pubchem.ncbi.nlm.nih.gov | |
Description | Data deposited in or computed by PubChem | |
Canonical SMILES |
CCN(CC)C(=O)OC1=CC=CC=C1 | |
Details | Computed by OEChem 2.3.0 (PubChem release 2021.05.07) | |
Source | PubChem | |
URL | https://pubchem.ncbi.nlm.nih.gov | |
Description | Data deposited in or computed by PubChem | |
Molecular Formula |
C11H15NO2 | |
Details | Computed by PubChem 2.1 (PubChem release 2021.05.07) | |
Source | PubChem | |
URL | https://pubchem.ncbi.nlm.nih.gov | |
Description | Data deposited in or computed by PubChem | |
DSSTOX Substance ID |
DTXSID50340827 | |
Record name | Phenyl diethylcarbamate | |
Source | EPA DSSTox | |
URL | https://comptox.epa.gov/dashboard/DTXSID50340827 | |
Description | DSSTox provides a high quality public chemistry resource for supporting improved predictive toxicology. | |
Molecular Weight |
193.24 g/mol | |
Details | Computed by PubChem 2.1 (PubChem release 2021.05.07) | |
Source | PubChem | |
URL | https://pubchem.ncbi.nlm.nih.gov | |
Description | Data deposited in or computed by PubChem | |
CAS No. |
65009-00-9 | |
Record name | Phenyl diethylcarbamate | |
Source | EPA DSSTox | |
URL | https://comptox.epa.gov/dashboard/DTXSID50340827 | |
Description | DSSTox provides a high quality public chemistry resource for supporting improved predictive toxicology. | |
Retrosynthesis Analysis
AI-Powered Synthesis Planning: Our tool employs the Template_relevance Pistachio, Template_relevance Bkms_metabolic, Template_relevance Pistachio_ringbreaker, Template_relevance Reaxys, Template_relevance Reaxys_biocatalysis model, leveraging a vast database of chemical reactions to predict feasible synthetic routes.
One-Step Synthesis Focus: Specifically designed for one-step synthesis, it provides concise and direct routes for your target compounds, streamlining the synthesis process.
Accurate Predictions: Utilizing the extensive PISTACHIO, BKMS_METABOLIC, PISTACHIO_RINGBREAKER, REAXYS, REAXYS_BIOCATALYSIS database, our tool offers high-accuracy predictions, reflecting the latest in chemical research and data.
Strategy Settings
Precursor scoring | Relevance Heuristic |
---|---|
Min. plausibility | 0.01 |
Model | Template_relevance |
Template Set | Pistachio/Bkms_metabolic/Pistachio_ringbreaker/Reaxys/Reaxys_biocatalysis |
Top-N result to add to graph | 6 |
Feasible Synthetic Routes
Descargo de responsabilidad e información sobre productos de investigación in vitro
Tenga en cuenta que todos los artículos e información de productos presentados en BenchChem están destinados únicamente con fines informativos. Los productos disponibles para la compra en BenchChem están diseñados específicamente para estudios in vitro, que se realizan fuera de organismos vivos. Los estudios in vitro, derivados del término latino "in vidrio", involucran experimentos realizados en entornos de laboratorio controlados utilizando células o tejidos. Es importante tener en cuenta que estos productos no se clasifican como medicamentos y no han recibido la aprobación de la FDA para la prevención, tratamiento o cura de ninguna condición médica, dolencia o enfermedad. Debemos enfatizar que cualquier forma de introducción corporal de estos productos en humanos o animales está estrictamente prohibida por ley. Es esencial adherirse a estas pautas para garantizar el cumplimiento de los estándares legales y éticos en la investigación y experimentación.